17 The Search for Life on Mars
Although it is dry and seemingly barren today, our next-door neighbor Mars may have once been a habitable planet. Finding any life on Mars, even a microbe, would represent a leap in our understanding of life in the universe, as it would mean that life existed in two separate locations in one solar system. Humans have been exploring the Red Planet with unmanned spacecraft since the 1960s and a great deal of evidence has emerged in support of a warmer and wetter Mars in the past. In this chapter, we review some of the key findings that point toward a once habitable Mars.
Learning Objectives
By the end of this chapter, you will be able to:
- Discuss findings from early missions in the 20th century to explore Mars
- Describe the terrain and geological features of Mars
- Discuss several lines of evidence that suggest Mars had liquid water on its surface in the past
- Describe the various rover space missions to Mars and what they have found so far
History and Early Missions
The possibility that Mars hosts, or has hosted, life has a rich history dating back to at least the middle of the nineteenth century. William Herschel observed Mars in 1783 and noted that Earth and Mars share some commonalities, such as having an atmosphere and going through seasonal cycles. By 1840, the length of a day on Mars was known to be very similar to that for the Earth, at just over 24 hours. These similarities between Earth and Mars fueled speculation that Mars, too, could harbor life. The Italian astronomer Giovanni Schiaparelli made detailed sketches of the Martian surface in 1877 and he labeled some straight line features as “canali,” an Italian word that can be translated to mean channels or canals, where channels are a natural geological feature and canals are manmade. That same year, 1877, American astronomer Asaph Hall discovered two small moons orbiting Mars; Hall named these moons Phobos and Deimos, meaning fear and panic, after the sons of Ares (the Greek counterpart of Mars).
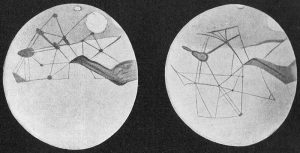
The American astronomer Percival Lowell, the brother of the poet Amy Lowell, was intrigued by the possibility of life on Mars suggested by Schiaparelli’s straight line “canali” features. Lowell, who came from a wealthy Boston family, built an observatory in Flagstaff, Arizona, called Lowell Observatory, in 1894 to undertake his observations of the surface of Mars. Lowell also published several books about the possibility of life on Mars, including Mars As The Abode Of Life and Mars And Its Canals. Not all scientists subscribed to Lowell’s view that Mars was inhabited, and in 1894 Edward Barnard made his own observations and did not see the straight line canals observed by Schiaparelli and Lowell. To add to the public’s imagination, H.G. Wells published his sci-fi novel The War of the Worlds in 1897.
The idea that Mars was inhabited by advanced life persisted throughout a good deal of the 20th century. The book Intelligent Life in the Universe, written by I.S. Shklovskii and Carl Sagan in 1966, includes a chapter dedicated to the possibility that the two moons of Mars are not natural but were artificially created by inhabitants of Mars. This was one year after the first images of Mars came in from the Mariner 4 mission. English artist David Bowie chimed in in 1971 with his hit song “Life on Mars?”. In 1976, prior to the Viking missions landing on Mars, Carl Sagan and Joshua Lederberg published the article “The prospects for life on Mars: A pre-Viking assessment” in the journal Icarus. In the abstract, they note:
“Large organisms, possibly detectable by the Viking lander cameras, are not only possible on Mars; they may be favored.”
Mariner and Viking Missions
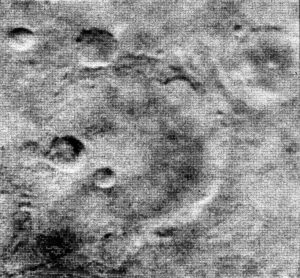
A global view of Mars emerged after images from the Mariner flyby and orbiter missions were transmitted back to Earth. The first visitor was the US Mariner 4, which flew past Mars in 1965 and transmitted 22 photos to Earth. These pictures showed an apparently bleak planet with abundant impact craters. In those days, craters were unexpected; some people who were romantically inclined still hoped to see canals or something like them. In any case, newspaper headlines sadly announced that Mars was a “dead planet.” In 1971, NASA’s Mariner 9 became the first spacecraft to orbit another planet, mapping the entire surface of Mars at a resolution of about 1 kilometer and discovering a great variety of geological features, including volcanoes, huge canyons, intricate layers on the polar caps, and channels that appeared to have been cut by running water. Mariner 9 also collected data about the Martian atmosphere using two spectrometers on board. Geologically, Mars didn’t look quite so dead after all. The data from Mariner 9 allowed the Viking 1 and 2 missions to launch in 1975 and successfully land on Mars in 1976.
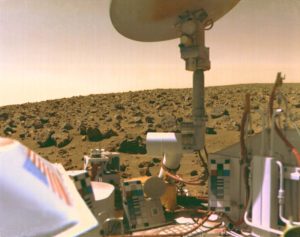
The Viking camera’s did not reveal any large organisms on Mars (unless they hopped on the cameras to avoid being seen). But it did show a compelling view of the rock-strewn rusty surface of Mars. The Viking landers landed in different regions of Mars: Viking 1 at Chryse Planitia and Viking 2 at Utopia Planitia. Both of these regions are fairly smooth plains in the northern lowlands of Mars and were chosen to help ensure a safe landing, but they also contain features that, from previous images, looked like they could be associated with water. The Viking missions contained the tools to perform soil experiments on Mars, with the goal of looking for evidence of life. These experiments are discussed in detail below.
Extraordinary Claims: The Face on Mars

People like human faces. We humans have developed great skill in recognizing people and interpreting facial expressions. We also have a tendency to see faces in many natural formations, from clouds to the man in the Moon. One of the curiosities that emerged from the Viking orbiters’ global mapping of Mars was the discovery of a strangely shaped mesa in the Cydonia region that resembled a human face. Despite later rumors of a cover-up, the “Face on Mars” was, in fact, recognized by Viking scientists and included in one of the early mission press releases. At the low resolution and oblique lighting under which the Viking image was obtained, the mile-wide mesa had something of a Sphinx-like appearance.
Unfortunately, a small band of individuals decided that this formation was an artificial, carved sculpture of a human face placed on Mars by an ancient civilization that thrived there hundreds of thousands of years ago. A band of “true believers” grew around the face and tried to deduce the nature of the “sculptors” who made it. This group also linked the face to a variety of other pseudoscientific phenomena such as crop circles (patterns in fields of grain, mostly in Britain, now known to be the work of pranksters).
Members of this group accused NASA of covering up evidence of intelligent life on Mars, and they received a great deal of help in publicizing their perspective from tabloid media. Some of the believers picketed the Jet Propulsion Laboratory at the time of the failure of the Mars Observer spacecraft, circulating stories that the “failure” of the Mars Observer was itself a fake, and that its true (secret) mission was to photograph the face.
The high-resolution Mars Observer camera (MOC) was reflown on the Mars Global Surveyor mission, which arrived at Mars in 1997. On April 5, 1998, in Orbit 220, the MOC obtained an oblique image of the face at a resolution of 4 meters per pixel, a factor-of-10 improvement in resolution over the Viking image. Another image in 2001 had even higher resolution. Immediately released by NASA, the new images showed a low mesa-like hill cut crossways by several roughly linear ridges and depressions, which were misidentified in the 1976 photo as the eyes and mouth of a face. Only with an enormous dose of imagination can any resemblance to a face be seen in the new images, demonstrating how dramatically our interpretation of geology can change with large improvements in resolution. The original and the higher resolution images can be seen in Figure 5 below.
After 20 years of promoting pseudoscientific interpretations and various conspiracy theories, can the “Face on Mars” believers now accept reality? Unfortunately, it does not seem so. They have accused NASA of faking the new picture. They also suggest that the secret mission of the Mars Observer included a nuclear bomb used to destroy the face before it could be photographed in greater detail by the Mars Global Surveyor.
Space scientists find these suggestions incredible. NASA is spending increasing sums for research on life in the universe, and a major objective of current and upcoming Mars missions is to search for evidence of past microbial life on Mars. Conclusive evidence of extraterrestrial life would be one of the great discoveries of science and incidentally might well lead to increased funding for NASA. The idea that NASA or other government agencies would (or could) mount a conspiracy to suppress such welcome evidence is truly bizarre.
Alas, the “Face on Mars” story is only one example of a whole series of conspiracy theories that are kept before the public by dedicated believers, by people out to make a fast buck, and by irresponsible media attention. Others include the “urban legend” that the Air Force has the bodies of extraterrestrials at a secret base, the widely circulated report that UFOs crashed near Roswell, New Mexico (actually it was a balloon carrying scientific instruments to find evidence of Soviet nuclear tests), or the notion that alien astronauts helped build the Egyptian pyramids and many other ancient monuments because our ancestors were too stupid to do it alone.
Geology of Mars
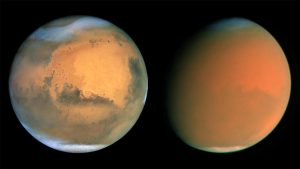
After the Viking missions concluded, Mars was not visited by spacecraft again until the 1990s. Beginning with the Mars Pathfinder and Mars Global Surveyor missions in 1997, there have been over a dozen successful missions to explore Mars in the past 25 years. Each mission has contributed in various ways to our global understanding of Mars as a planet. Several missions, which we highlight in the next section, have had a specific goal of assessing the habitability of Mars or looking for signs of life.
The map below (Figure 7) was created using an altimeter — an instrument that uses lasers to measure the altitude (or height) of different parts of the surface — onboard the Mars Global Surveyor. The map is color-coded by the height of the surface, where the yellow regions are “ground level” at 0 km, blue/purple regions are at depths of about 5-7 km, and the grey/white regions have the highest altitudes, at over 12 km high for the white circular regions (these white circles are the tops of volcanoes). The Martian volcano Olympus Mons stands at a height of over 22 km, which is more than twice the height of Earth’s tallest mountain from base to peak (Mauna Kea in Hawaii, part of which is under water), making Olympus Mons the tallest known mountain in the solar system.
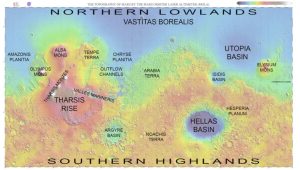
Other notable features are seen on the Martian surface, such as the Valles Marineras (Mariner Valleys, named for the Mariner 9 mission which first revealed them). Valles Marineras is a canyon system that extend for about 5000 kilometers (nearly a quarter of the way around Mars) along the slopes of the Tharsis bulge. If it were on Earth, this canyon system would stretch all the way from Los Angeles to Washington, DC.
Concept Check: the Surface of Mars
Looking at the topographic map above of Mars (Figure 7), which regions are at the highest altitudes (elevation) and which regions are at the lowest altitudes? What do you think created Hellas Basin?
Can you find the Chryse and Utopia Planitia, the landing sites for Viking 1 and 2? Are these regions as deep as the Hellas Basin?
To further explore the terrain of Mars, you can load up Google Mars! Go to https://www.google.com/mars/ and start exploring. For example, you can click on “Spacecraft” at the top and see the locations of different Martian landers and rovers.
Globally, Mars has two distinct regions: the heavily cratered highlands in the southern hemisphere and the smoother low-lying volcanic plains (the “lowlands”) in the northern hemisphere. The Moon also has similar surface geology, with the smoother plains (the lunar mare) representing a younger surface that was “re-paved” by lava around 3.5 billion years ago. Based on counting the numbers of craters in different regions and analysis of layered rocks, the geological history of Mars can be broken down into three eons: the Noachian, the Hesperian, and the Amazonian.
General Properties of Mars
We can gain some insight into the current conditions on Mars by considering its size and distance from the Sun. Mars is smaller than the Earth, with a radius about half that of the Earth and a mass of about 10% that of the Earth. The density of Mars is 3.9 g/cm3 (Earth’s density is 5.5 g/cm3), which suggests a composition consisting primarily of silicates but with a small metal core. The smaller size of Mars compared to Earth means that Mars loses its internal heat more rapidly than the Earth, since the cooling rate depends on the radius. Mars has a surface gravity that is just 38% the value of Earth’s surface gravity, meaning that material can escape more easily from Mars’ gravitational pull. Finally, we note that Mars is about 50% further away from the Sun than the Earth is, meaning that Mars receives less energy from the Sun. Venus is closer to the Sun than Earth and consequently experienced a runaway greenhouse effect. Mars, on the other hand, experienced a runaway “icehouse” effect.
Today, Mars has lost almost all of its original atmosphere because of its low surface gravity and stripping by the solar wind (a stream of highly energetic particles emitted by the Sun). Mars also no longer has a protective magnetosphere so, if any life did currently exist on Mars, it would be challenging for it to survive in a high-radiation environment, which includes cosmic rays.
The Search for Life
In the quest to find life on Mars, either in the past or present, we can look evidence of liquid water and for the essential elements that make up all life on Earth — CHNOPS. Given the commonalities between Mars and Earth noted earlier, such as a similar cycle of seasons and surface geology, we might expect that life could have formed in the same manner around the same time on early Mars as on Earth if there was liquid water in the past.
The Viking Biology Experiments
The earliest missions to Mars provided some hints that liquid water—one of life’s primary requirements—may once have flowed on the surface, and later missions have strengthened this conclusion. The NASA Viking landers, whose purpose was to search directly for evidence of life on Mars, arrived on Mars in 1976.
There were four different experiments that the Viking landers were equipped to conduct to look for byproducts of life in the Martian soil. The experiments were: gas-exchange (GEX), pyrolitic release (PR), labeled release (LR) and the gas chromatograph mass spectrometer (GCMS). The GEX and LR experiments both worked by adding nutrients to the soil and then attempting to detect gases released by organisms that consumed the nutrients. The PR experiment simulated the early Martian atmosphere and looked for signs of photosynthetic organisms. GCMS was slightly different in that its goal was to look for organic materials in the soil. Of the four experiments, only the LR produced positive results. However, the LR results have generally been deemed insufficient evidence for life since the GCMS experiments did not find any organics in the soil. However, some of the scientists who designed the LR experiment still interpret the results as supporting life. Extraordinary claims…
Viking’s onboard instruments found no organic molecules (the stuff of which life is made), and no evidence of biological activity in the martian soils it analyzed. The lack of any biological activity in the Martian soil is not particularly surprising because, despite the evidence of flowing liquid water in the past, liquid water on the surface of Mars is generally not stable today. Over much of the Martian surface, temperatures and pressures are so low that pure water would either freeze or boil away (under very low pressures, water will boil at a much lower temperature than usual). To make matters worse, unlike Earth, Mars does not have a magnetic field and ozone layer to protect the surface from harmful solar ultraviolet radiation and energetic particles. However, Viking’s analyses of the soil said nothing about whether life may have existed in Mars’ distant past, when liquid water was more abundant. Water in the form of ice exists in abundance on Mars, not too deep beneath its surface (for example, at the ice caps). Water vapor is also a constituent of the atmosphere of Mars.
Surface Features
Early evidence for surface features shaped by the presence of water came from the Mariner 9 mission, including the Valles Marineris, ancient river channels, tear-drop shaped islands and the presence of ice caps. Mariner 9 observed valley networks and outflow channels. Figure 8 below shows valleys and channels taken by the Viking Orbiter and the Mars Global Surveyor.
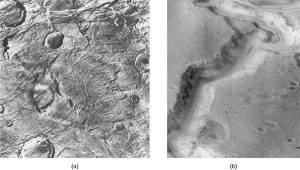
The camera aboard the Mars Global Surveyor provided high-resolution (down to a few meters, which is good enough to see a small truck or bus) images of gullies on Mars. The gullies were found on the steep walls of valleys and craters at high latitudes and appear to be carved by flowing water. These gullies are very young: not only are there no superimposed impact craters, but in some instances, the gullies seem to cut across recent wind-deposited dunes. Perhaps there is liquid water underground that can occasionally break out to produce short-lived surface flows before the water can freeze or evaporate. The Mars Reconnaissance Orbiter, which arrived at Mars in 2006 and is still active today, also took high resolution images of Martian gullies.
Aqueous Minerals
One way to find signs of past water on Mars is to look for minerals that can only form in the presence of water. These are known as aqueous minerals (the word aqueous comes from the Latin word aqua, meaning water) and there are several different types.
Clay minerals are found in sediments on the sea floor on Earth, and clays have been suggested as the original site for the first life on Earth. They have also been found in a few different locations on Mars, such as the Nili Fossae region.
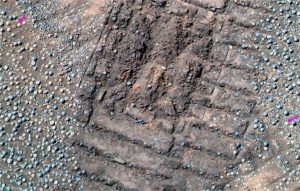
In 2004, the Opportunity rover found jarosite and hematite at Meridiani Planum on Mars; these are both minerals that form in a watery environment. Jarosite is a sulfate that can indicate interactions with acidic water. The hematite was found in the form of small spheres that resemble blueberries and as such have taken on this descriptive name. Figure 9 to the right shows blueberries on Mars seen next to the Opportunity rover’s tracks.
The Curiosity rover landed on Mars in 2012 and has now been there for over 4,000 sols (a sol is a Martian day, which is slightly longer than an Earth day). Curiosity landed in Gale Crater, which was selected as the landing site because of evidence that there were clay minerals and sulfates in the sediments on Mt. Sharp. The video below provides some background on Gale Crater and shows Mt. Sharp at its center. Curiosity has been climbing up Mt. Sharp and studying the composition of its layers. As noted in the video clip, the geological layers starting from the bottom provide a way of looking back at the history of the crater and are especially important in understanding when water existed inside the crater in the past.
Guide to Gale Crater
Video Credit: @NASAJPL
The strongest evidence of an ancient habitable environment came from analyzing a very fine-grained rock called a mudstone—a rock type that is widespread on Earth but was unknown on Mars until Curiosity found it. The mudstone can tell us a great deal about the wet environments in which they formed. Curiosity has an instrument called CheMin (short for Chemistry and Mineralogy) on board the rover that can analyze soil and rocks and identify what minerals, and in what quantity, are present. The rover first collects a sample and then deposits it into CheMin for analysis. Figure 10 shows the results for ten sites where samples were collected by Curiosity, starting at the bottom of Gale Crater (Yellowknife Bay) and subsequently as the rover moved up Mt. Sharp. For each site, a pie chart shows what minerals were found. The legend shows the colors used for the pie charts — notice that clay minerals, jarosite and hematite are all found, and their amounts vary as the rover climbs up the mountain. This provides not only confirmation of past liquid water on Mars but also a detailed look back at how the water varied (for example, the clay minerals appear at Yellowknife Bay and then reappear as the rover reaches Murray Buttes).
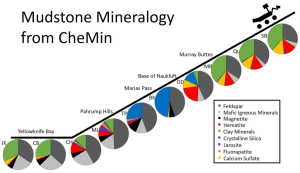
Ice
There is water on Mars today but it is in the form of ice. This alone is significant, as it is easy to imagine that some of this ice existed as liquid water when Mars had a warmer environment. Today, ice is found at the polar ice caps, inside craters and below the surface. The Phoenix Mission directly detected water ice on Mars in 2008 (Figure 11). The Phoenix spacecraft landed near the north polar cap in summer. Controllers knew that it would not be able to survive a polar winter, but directly measuring the characteristics of the polar region was deemed important enough to send a dedicated mission. The most exciting discovery came when the spacecraft tried to dig a shallow trench under the spacecraft. When the overlying dust was stripped off, they saw bright white material, apparently some kind of ice. From the way this ice sublimated over the next few days, it was clear that it was frozen water. (Sublimation is the process of a solid turning directly into gas when it is heated)
Organic Compounds
Evidence of liquid water flowing in the past clearly points to Mars once being a habitable planet, albeit more than 3 billion years ago. But life as we know it also needs certain chemical elements that are essential to building amino acids, proteins and DNA. The basic building blocks for life are carbon, hydrogen, oxygen, nitrogen, phosphorous and sulfur (CHNOPS). The Perseverance rover, which landed on the surface of Mars in July of 2021 (two other Mars missions, the UAE’s Hope orbiter and China’s Tianwen 1 mission, also reached Mars around the same time) is taking the search for life on Mars one step further than the Curiosity rover. Curiosity’s main goal was to establish if Mars once was habitable, and much evidence from the Curiosity rover described above supports this claim. Perseverance is looking for evidence of potential biosignatures on Mars. If any biosignatures — meaning signs of life — are found by Perseverance, they will still need to be analyzed in great detail to rule out the possibility that they formed naturally rather than by life.
Concept Check
Go to the Mars rock samples site at https://mars.nasa.gov/mars-rock-samples and pick one of samples to study. Describe what was collected and how it may contribute to our understanding of life on Mars.
The Perseverance mission is unique — it is the first Mars mission to collect samples that will eventually be sent back to Earth for analysis and it also has a helicopter, named Ingenuity! Perseverance is collecting rock and soil samples as it moves around the crater and visits areas such as an ancient river delta. The samples are sealed into tubes and these will eventually will be picked up by a future mission to Mars; some estimates put the sample return date to Earth in the 2030s.
Perseverance has an instrument appropriately named SHERLOC (the H in SHERLOC stands for Habitable — Scanning Habitable Environments with Raman & Luminescence for Organics & Chemicals) that can detect the presence of organic molecules, as well as aqueous minerals. SHERLOC detected organics in Jezero crater, where the rover is exploring. The Curiosity rover also found organics in Gale crater, but the Perseverance detections have added value as they can be correlated with minerals inside the rocks.
The video clip below gives a tour of Jezero Crater, where Perseverance landed and has been collecting rock and soil samples. In the video, notice that there are mudstones in Jezero crater, just as in the Gale crater that the Curiosity rover is exploring.
Prospects for Life
Five decades of robotic exploration have allowed us to develop a picture of how Mars evolved through time. Early Mars had epochs of warmer and wetter conditions that would have been conducive to life at the surface. However, Mars eventually lost much of its early atmosphere and the surface water began to dry up. As that happened, the ever-shrinking reservoirs of liquid water on the martian surface became saltier and more acidic, until the surface finally had no significant liquid water and was bathed in harsh solar radiation. The surface thus became uninhabitable, but this might not be the case for the planet overall.
Reservoirs of ice and liquid water could still exist underground, where pressure and temperature conditions make it stable. There is recent evidence to suggest that liquid water (probably very salty water) can occasionally (and briefly) flow on the surface even today. Thus, Mars might even have habitable conditions in the present day, but of a much different sort than we normally think of on Earth.
Our study of Mars reveals a planet with a fascinating history—one that saw its ability to host surface life dwindle billions of years ago, but perhaps allowing life to adapt and survive in favorable environmental niches. Even if life did not survive, we expect that we might find evidence of life if it ever took hold on Mars. If it is there, it is hidden in the crust, and we are still learning how best to decipher that evidence.
Want to know more: Rare Earth?
-
With the discovery of planets orbiting other stars in the 1990s, enthusiasm was mounting for the search for life on other worlds. In 2000, a popular book, “Rare Earth,” by Ward and Brownlee, suggested that primitive life is very common but technological life is rare. Without a single other example of life beyond Earth, the book spurred a lot of discussion among scientists and the interested public.
The main premise of the book was that too many random, chance conditions were required for homosapiens to evolve. In addition, once our technological species appeared, there were new threats to the survival of life (nuclear war, climate change, over-population). Some of the essential conditions and circumstances identified by Brownlee and Ward are listed in the Table below.
Essential Characteristic Reasoning Right mass planet Too low mass and the planet could not hang on to its atmosphere; too high mass and the high surface pressure would impede evolution of animals. Right mass star Too high mass and the star would have a very short lifetime and emit most of its energy at damaging ultraviolet wavelengths; too low mass and strong magnetic activity from the star would strip away the planet atmosphere. Habitable zone orbit Too close and the planet would experience runaway greenhouse warming, like Venus; too far and liquid water would be frozen and biochemical reaction rates would be sluggish. Atmosphere An atmosphere is needed to protect life from ultraviolet radiation. Oxygen The invention of photosynthesis was required to produce atmospheric oxygen for efficient aerobic metabolisms. Microbes could be successful organisms in anaerobic conditions, but not complex eukaryotes. Liquid water Some surface water, but not so much as to cover all of the land (hard for technology to develop under water). Plate tectonics To participate in a negative feedback loop and stabilize the climate. Global magnetic field Without a global magnetic field, the wind of charged particles from the surface of the host star will strip away the atmosphere. Large moon To stabilize the tilt of the planet. Mars-like world So prebiotic chemistry and life could get a head start. Gas Giant outer planet To act as a sink for incoming comets. Right location in the galaxy Too close to the galactic center and high energy radiation from massive stars would be a threat; too far out in the galactic disk and there would be less chemical enrichment. Mass extinctions Some mass extinctions to allow for biodiversity, but not too many. The counter point made by many scientists was that the argument was too anthropomorphic. Some characteristics make sense (right mass planet, atmosphere, water) but others seem less relevant, for a few different reasons:
Not a bottleneck: The circumstances that allowed life on our planet might not be a requirement for life elsewhere. Some characteristics do not present a bottleneck. There are hundreds of billions of “right mass stars,” and very few OB stars with short lives and peak energy emission of damaging ultraviolet radiation. It now appears that there are also billions of planets similar in mass to the Earth and orbiting in the habitable zone. Many of these stars and planets reside in intermediate regions in the galactic disk.
Redundant: Some of the characteristics are interrelated. In the same way that there is redundancy in the requirement: “you have to have a tree and it has to have leaves and it has to have a trunk and branches,” some of the characteristics follow “for free” from others. A large subset of Earth-like planets in the habitable zone will have liquid water, plate tectonics, and global magnetic fields. They will naturally outgas or accrete atmospheres.
Uncertain impact: Other factors in Ward & Brownlee’s list have an uncertain impact. Did we really need Mars to jump-start life on Earth? If we didn’t have Jupiter, would there be fewer objects trapped in the asteroid belt? Would a few more impacts have been a good thing for life on Earth? Does it matter if the obliquity of the Earth precesses at a faster or slower rate?
Mass extinctions fall on the spectrum of natural selection, which must be fundamental for life. It does seem likely that simple microbial life will be far more common than complex organisms. There is likely a pyramid scheme for life, with single cell organisms at the bottom, and complex organisms like whales, velociraptors, and humans near the top. This is exactly what astrobiologists are trying to learn.
Key Concepts and Summary
Craters on the surface of small bodies like Mars show that erosive processes no longer exist on these objects. The small mass and size of Mars means that this planet has lost most of its atmosphere. However, features on the surface of Mars suggest that this planet once had bodies of surface water, making one of our closest neighbors a key target for exploration with NASA missions. NASA rovers dig beneath the surface and analyze Martian soil for signs of biochemical processes or even simple cellular life.
Review Questions
Summary Questions
- When did the first dedicated experiments to search for life on Mars begin? Discuss some of the ideas about life on Mars that predated these searches.
- What are the main properties of the planet Mars (for example, its density and surface gravity)? How do these properties relate to the possible habitability of Mars?
- How is the geology of Mars similar to that on Earth? How does it differ?
- What molecules make up the atmosphere of Mars?
- Does Mars have a magnetic field today, and did it have one in the past? Explain your answer.
- What are aqueous minerals? How can their presence point toward the conditions for life on Mars?