26 The Drake Equation and the Fermi Paradox
Is anybody out there?
Learning Objectives
By the end of this chapter, you will be able to:
- Explain why the Drake Equation was formulated and what it seeks to estimate
- Describe the main factors that go into the Drake Equation
- Discuss the “Fermi Paradox” and some reasons reasons why no other life has been detected
- Come up with and justify your own opinion on whether or not there is other life in the cosmos
The Drake Equation
In 1961, just one year after Frank Drake’s Project Ozma was completed, a conference was held at the Green Bank Observatory in West Virginia on the topic of “Extraterrestrial Intelligent Life,” specifically if the ongoing searches for extraterrestrial intelligence had any chance of actually detecting a real ET signal. A small number of experts were brought together for the meeting, including Carl Sagan, who was just 27 at the time, and John Lilly, who studied dolphin language and communication. Because of Lilly’s expertise, the group called themselves “The Order of the Dolphin.” To help organize an agenda for the meeting, Drake put together an equation to estimate how many intelligent civilizations should exist in the Milky Way galaxy; the equation contained several terms that Drake felt were key to considering this question.
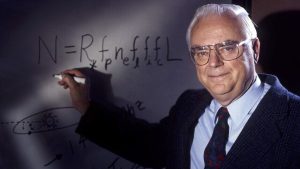
The equation, which is presented below, provided enough discussion points for the entire three-day conference and remains a useful heuristic tool in discussions on the possibility of other life in the universe. Seth Shostak at the SETI institute characterizes the Drake equation as a map, not a destination. The equation is made up of seven different terms that can be multiplied together to provide an estimate of the number of civilizations that we should expect to hear from. The terms are fractions and their values can range from 0 to 1, where 1 is 100%.
where
is the number of communicative civilizations that we could detect
is the rate of formation of stars like the Sun in our Galaxy
is the fraction of stars with planets
is the number of these planets that have the right conditions for life
is the fraction of these planets where life develops
is the fraction of planets with life where intelligence emerges
is the fraction of intelligent life that develops communicating technology
is the lifetime of communicating civilizations.
The video below by SETI pioneer Jill Tarter summarizes the terms in the Drake Equation and makes some points worth keeping in mind as we consider the various terms. As she points out, the term is often considered the most important one to consider in making this estimation. She also reiterates that there is no “right answer” to the Drake equation, unlike other scientific equations that you have used.
After watching the video above, consider these questions:
- How could we justify approximating the Drake Equation to be
?
- Can we ever have a definitive answer to the Drake Equation? Explain why or why not.
Unless SETI succeeds or we can show that the Earth is the only life out there, remains uncertain.
As the attendees of the Green Bank conference did in 1961, let’s consider each term separately and then attempt to come up with an estimation. We will provide some provisional numbers, but many of them are merely educated guesses (some more educated than others).
Stars
We can make a rough estimation of the star formation rate of the galaxy, , by considering what we know about the galaxy today. Currently, the galaxy has on the order of 100 billion stars. We also know the galaxy to be approximately 10 billion years old. From this, we find that on average stars have been be forming at a rate of 10 stars per year.
= (100 billion stars)/(10 billion years) = 10 stars per year
This is a very simplistic estimate. It does not take into account how many of these stars may be suitable for life, such as short-lived O or B type stars, or whether the rate of star formation has been constant over the lifetime of the galaxy. However, this value gives a well-educated estimate. A more conservative estimate that takes into account the different types of stars and how often they form leads to a lower number of = 1 star per year.
Planets
Thank to discoveries over the past decade, we now have a good estimate for the fraction of stars with planets. This was completely unknown at the time Frank Drake first wrote down his equation — recall that the first exoplanet was not detected until the 1990s. The discoveries from the Kepler mission provide statistical evidence that essentially every star has one or more planets. When you look up at the night sky, there are actually more unseen exoplanets there than there are stars. We can therefore assign a value of 1 for .
Earth-like planets
The next parameter, , is the number of planets that have the conditions for life to develop. This brings to mind the concept of the habitable zone (HZ) around a star — if we can quantify how many rocky planets typically fall into the HZ around their star, this would be a good starting point. However, we need to keep in mind that some of the most promising places to find life in our solar system are the moons Europa, Enceladus, and Titan, all of which are not in the Sun’s HZ. Also, just because a planet falls into the HZ around its star does not mean it can support life; factors such as flares and tidal locking can impact the potential habitability of a planet. Astronomers have nonetheless used Kepler data to extrapolate out from the parameter space where Earth-sized planets were detected and estimate that the number of habitable Earths ranges from 0.5 to 3 per system.
Life
The term asks us to consider the fraction of Earth-like planets in the habitable zone where life of any kind (including single-celled microbes) emerges. This term must be non-zero, because there exists life on Earth but we have yet to find life anywhere else. What we do know is that the Earth formed about 4.56 billion years ago. We have firm evidence of life from stromatolites at 3.5 billion years ago, which must have been preceded by less complex organisms. These numbers indicate that life formed relatively quickly on Earth, even though it is not yet clear to us how. If we invoke the Copernican Principle here, then Earth should not be special in this regard, but we must contend that we just don’t know. We can also take the results of Miller-Urey-type experiments as evidence in favor of life developing naturally given the right conditions, but those results did not find life itself, just the building blocks (amino acids).
We can infer that perhaps life appears between 10% and 100% of the time on habitable planets, but you should make your own guess for this fraction. What is your justification?
How do we know: what will intelligent signals look like?
Given the difficulties we have had defining “life”, it is perhaps no surprise that there are even more difficulties defining “intelligence”. Many assumptions have been made about what intelligence implies and how it can be measured which are increasingly criticized by social scientists and philosophers. One of the first steps that we take to try to define intelligence is to distinguish between lifeforms that are not intelligent and those that are, but attempts to make these clean distinctions have run into problems. For example, some attempts at defining human intelligence as distinct from other lifeforms invoked consciousness or sentience (roughly knowledge of one’s self identity) as a criteria. This requires, however, a theory of mind that assumes these aspects of our existence are generalizable and identifiable in other life forms in ways that are difficult to measure. While neuroscientists are actively investigating consciousness in new and clever ways, there is no foolproof way to determine whether and how any given lifeform is conscious at this time. Using that as a criteria for intelligence, then, will possibly cause more problems than it will solve.
At one time it was assumed that what distinguished human intelligence from that of other animals was an ability to communicate through language. Nevertheless, anyone who has spent significant time with a dog knows that animals are capable of communication in very sophisticated ways. The late Koko the Gorilla had a sign-language vocabulary in excess of 1000 signs by some measures, although whether that constituted learning a language and to what extent her human handlers “projected” communication onto Koko’s interactions is of enough concern to call into serious question the claims that she “learned” sign language. Controversies over intelligence and, in particular, psychometric testing of human beings, have also highlighted the problem of under what research contexts different definitions for intelligence in individuals are useful. IQ tests, for example, may highlight more about what is valued in a particular societal contexts instead of probing some manner of objective measure of whom is more intelligent than whom.
What those interested in the Search for Extra-Terrestrial Intelligence (SETI) use as a definition for intelligence is different from that which human or biological researchers in the subject use. Astronomers assume that the ability to produce similar technology to our own to enable interstellar communication is the mark of a group of intelligent lifeforms. As far as we know, the only lifeforms capable of such on Earth are humans. Even if a particular individual human does not know how to construct interstellar communication themselves, the knowledge and the skills were possible for us as a group to develop and create as demonstrated by the existence of the technology itself. Evolutionary biologists still debate when humanity first had the intellectual capacity to develop the technology of our modern world, but most would agree that early humans from at least 100,000 years ago exhibited the same brain-size, tool-making abilities, and language processing that we have today and that seem to be necessary to accomplish this. Research is ongoing in attempts to answer the question of how smart our ancestors were in comparison to us which may have implications for how we ask these questions in the first place.
But why should interstellar communication be the hallmark of intelligence?
If we want to answer the question “Are we alone?” we need to find a way to discover the other intelligent lifeforms. If any intelligent, technical societies have arisen, as has happened on Earth in the most recent blink of cosmic time, how could we make contact with them?
This problem is similar to making contact with people who live in a remote part of Earth. If students in the United States want to converse with students in Australia, for example, they have two choices. Either one group gets on an airplane and travels to meet the other, or they communicate by sending a message remotely. Given how expensive airline tickets are, most students would probably select the message route.
In the same way, if we want to get in touch with intelligent life around other stars, we can travel, or we can try to exchange messages. Because of the great distances involved, interstellar space travel would be very slow and prohibitively expensive. The fastest spacecraft the human species has built so far would take almost 80,000 years to get to the nearest star. While we could certainly design a faster craft, the more quickly we require it to travel, the greater the energy cost involved. To reach neighboring stars in less than a human life span, we would have to travel close to the speed of light. In that case, however, the expense would become truly astronomical.
Intelligence
As we saw above when considering the fraction of habitable planets that go on to develop life, the estimates get increasingly difficult as we move into the biological factors ( and
) in the Drake Equation. As emphasized in the box above on “How do we know what intelligence is?”, it is hard to describe, hard to quantify, and hard to detect intelligence. Besides the example of intelligent life on Earth, we have no other concrete evidence towards a true value for the fraction of life that becomes intelligent. We could again use what happened on Earth as a general guiding example – a sort of Copernican Principle view for biology. From this viewpoint, Earth is not special and any life that forms should eventually develop intelligence.
One way to approach the question of intelligence developing is to ask when intelligent life first appeared on Earth. We know that complex life on Earth requires energy, which requires efficient metabolism. On Earth this takes the form of aerobic respiration, and the Cambrian explosion, about 540 million years ago, marks the time when complex life emerged. If we take 4 billion years ago as the time when the first life appeared on Earth, it then took 3.5 billion years for that life to evolve to be complex enough for intelligence. We can then estimate as
= (0.5 billion years)/(4 billion years) = 0.125
Inherently, right or wrong, we are guessing that life everywhere will evolve and that statistically perhaps 13% of the habitable planets that we find with life will have life forms that evolved to become intelligent.
However, we have no true understanding of how typical our planet is. We do not have a sense of whether the evolution of complex life is rare or inevitable for all Earth analogs. An upper bound might be 1, meaning all planets with life will eventually evolve the complexity that leads to intelligence or it might happen on one of a million inhabited worlds. That’s a big range!
Technology
The next term, , tries to capture another attribute that is hard to estimate. On inhabited planets with intelligent life, how often will that life develop technology so that it can communicate across the galaxy? We are technological adolescents — intelligent life on Earth has had communication technology for only about 100 years. That is a tiny fraction of time for intelligent life on Earth: 100 years out of 200,000 years that our species, Homo sapiens, have existed.
While we can easily identify other species on Earth that are intelligent, such as dolphins, bats and cats, it is a stretch to imagine any of these other intelligent species developing the capability for interstellar communication, suggesting a low probability for . A familiar argument by now in favor of a higher probability is the Copernican Principle, but this argument must be tempered with the reality that we simply do not have enough data to make any meaningful estimates of
.
Lifetime of an Advanced Civilization
Once a civilization has become advanced enough to develop science and the technology to build radio telescopes and transmitters, how long will it last? In the discussion of above, we noted that Earth has only been in this category for 100 years. Technology has rapidly advanced in that century, sometimes in ways to extend the favorable conditions for life on Earth and other times in ways that put the longevity of our planet at great risk. Biotechnology and genetic engineering are at a stage where we can combat and eradicate many diseases. On the other hand, the average temperature of the Earth is increasing exponentially and habitats are being destroyed as a result and some species need to relocate to avoid extinction. AI is now commonplace and this is also seen by some as positive and others as detrimental to the survivability of humanity.
The global political situation in 1961, when the Drake Equation was first presented, was tense as the Cold War put the Earth at increased risk for nuclear destruction. The risk of catastrophic warfare still looms more than 50 years later.
Considering these factors, a pessimistic take on would be maybe 100 more years or less.
Estimating N
Finally, the finale. We can collect our musings from above to calculate a value for N, the number of communicating civilizations that we could detect. Gather all of your values for each term in the Drake Equation and then use the calculator in the box below (or simply carry out the math on your own — it is just multiplication) to get your value of .
Once you have all of your values ready, you can use the Drake Equation calculator provided by PBS: https://www.pbs.org/lifebeyondearth/listening/drake.html
The Fermi Paradox
“Where is everybody?”
Physicist Enrico Fermi is credited with posing this question in 1950 during lunch with some colleagues — all having worked on the Manhattan Project with Fermi — at Los Alamos National Lab in New Mexico. There had been a recent increase in reports of UFO sightings and this led Fermi to pose the question and ponder why we haven’t heard from any other extraterrestrial civilizations. The question is not so much a paradox per se but a prompt for discussions on the challenges of detecting other life in the cosmos. The question becomes a paradox if we conclude that the universe is filled with life…and we should see it everywhere but don’t.
After considering the various terms in the Drake Equation, we have some context to think about reasons why we have not made contact. Of course, one easy solution is that we truly are alone and the only instance of life in the universe. While a generally sobering thought to contemplate, we must acknowledge that this is a possibility.
They’re Out There
Let’s consider the Fermi Paradox if we assume that other intelligent extraterrestrial civilizations exist in our galaxy. You can keep in mind your estimate of the number of such civilizations from the Drake Equation above as we make this assumption (for example, if you estimated a very low value for , then you may consider this a poor assumption). If other advanced life is out there and this life developed much earlier than our technological civilization on Earth, we might expect that the galaxy would be completely filled with evidence of this more advanced civilization. For example, they could have sent out self-replicating probes to all of the planets in the galaxy, either to see what’s there or to mine resources.
Before moving on and reading a few possible resolutions, take some time to think of your own resolutions to the Fermi Paradox in this case, as suggested in the box below.
Assume that there are a large enough number of other technologically advanced civilizations in the galaxy that we should expect to have heard from at least one of them by now.
Make a list of reasons as to why we have not heard from these civilizations yet (or may never hear from them).
We Don’t Recognize Them
One possibility contends that other advanced life may not communicate in the same ways that we do on Earth. Technology on Earth has exploded in the century since the first radio transmissions were sent out, and it is seems possible (as AI flourishes) that our communication tools will continue to advance exponentially. If another civilization has a few billion years jump start on technology, they may be communicating in a way that we simply don’t recognize. Consider a less advanced but still intelligent species on Earth, such as bats. We simply cannot communicate in any meaningful way with bats about human society, and the world as experienced by a bat is extremely limited when compared to a human’s understanding of the cosmos. A similar argument holds that life outside of the Earth may develop and evolve completely differently than it did on Earth, perhaps based on an element other than carbon, and we simply are unequipped to perceive it. For example, life on Saturn’s moon Titan could use methane or ethane instead of water as the liquid to facilitate chemical reactions inside of cells.
Interstellar Travel Is Too Difficult
It is easy to assume that a civilization that is much more advanced than our own would have figured out how travel to other civilizations or, at the least, to be able to communicate their presence effectively. But what if this assumption simply does not hold up? While we currently have the technology on Earth to send a probe to the nearest star in our life time (Breakthrough Starshot’s design for a light sail to be sent to Proxima Centauri), this still does not get us very far. Perhaps this is a barrier that few or no civilizations will be able to overcome. This idea that there is a barrier that any life must overcome before reaching an advanced stage, such as a Type II or Type III civilization on the Kardashev scale, falls into a general category of ideas that imagine there is a “great filter” (or filters) that life must overcome before it can continue to evolve. If this evolutionary bottleneck still lies in the future for humans, we can imagine a very pessimistic outlook for our species evolving further and developing more advanced technology.
Even without traveling to another planet, two-way communication is always restricted by the speed of light. If a civilization just 100 light years away from Earth sends us a message that we receive and can interpret, we would need to wait at least 200 years for a response. A wormhole — an interstellar or even intergalactic portal — could allay these time scales, but there is no evidence that wormholes exist.
Some additional ideas on resolving the Fermi Paradox are discussed in the video below.
Are We Alone?
The Drake Equation and the Fermi Paradox both provide ways to narrow the answer to this question based on the astronomical and biological data in hand. In 1966, when Sagan and Shlovskii published their book Intelligent Life in the Universe, no exoplanets or protoplanetary disks had been detected and was unconstrained. Today, we can say with confidence based on evidence from the Kepler and TESS missions that
is close to 1. We have more data today to support the first three terms of the Drake Equation —
,
,
and — but the biological terms remain just as uncertain today as in 1966. Sagan estimated
to be 106, or 1 million, in the 1960s and he acknowledged that the most sensitive term is
, the lifetime of a technologically advanced civilization. He looked at
both optimistically and pessimistically and then took the average to arrive at the value of
other civilizations out there. This same guesstimate holds today.
We have not detected any evidence of other life in the universe. However, we are currently poised to be able to make a meaningful statement of whether or not other life is out there in the next decade. This is for two main reasons. First, SETI is now exploring more stars at the widest range of frequencies ever thanks to the Breakthrough Listen initiative. The sheer number and volume of stars surveyed by Breakthrough Listen should yield a statistically significant sample of results to draw meaningful conclusions from. Second, the James Webb Space Telescope allows us to point directly at a potentially inhabited planetary and study its atmosphere for biosignatures and technosignatures. Next-generation telescopes like the Habitable Worlds Observatory will allow Earth-sized exoplanets to be imaged.
Key Concepts and Summary
The Drake equation is a way of estimating the probability that life exists elsewhere. First written down by Frank Drake in 1961, the Drake equation includes factors like the rate of star formation, the number of planets per stars, the fraction of those planets with the right conditions for life to emerge and evolve into intelligent, technological civilizations. The final term is the lifetime of those communicating civilizations – a recognition that species and technology are ever changing and not permanent. With all of the searches that have been carried out, the Fermi paradox asks why we have not found anything yet: Where are they?