Optional Supplementary Chapter: History of Astrobiology
The cosmos is vast. To contemplate the mere existence of life in the universe, we must step back and ask some fundamental questions: What is the cosmos made of? What is at the center of the cosmos, if there is a center at all? Ancient Greek schools of thought marked a turning point in the approach to answering questions of this kind. Rather than invoking supernatural explanations to celestial phenomena, ancient Greek thinkers constructed empirical models to explain the universe.
Learning Objectives
By the end of this chapter, you will be able to:
- Discuss the different views of the cosmos expressed by Atomists and Aristotelians
- Understand the concept of models and describe the geocentric and heliocentric models
- Explain the apparent retrograde motion of planets
- Describe the significance of Galileo’s telescope observations
- Discuss why Galileo was accused of heresy by the Church in the 17th century
Ancient Greek Cosmologies
Our concept of the cosmos — its basic structure and origin —is called cosmology, a word with Greek roots (the Greek word kosmos means world). Before the invention of telescopes, humans had to depend on the simple evidence of their senses for a picture of the universe. The ancients developed cosmologies that combined their direct view of the heavens with a rich variety of philosophical and religious symbolism. Two main schools of thought regarding the nature of matter arose in the era of the ancient Greeks: the atomists and the Aristotelians. In the context of other life in the universe, the Aristotelians believed that the Earth was the only world and was at the center of the cosmos, while the atomists believed that many worlds can exist throughout the cosmos, and some of these worlds can naturally have life.
What is the Universe made of?
In the sixth century BCE, Greek thinkers are documented to have been arguing over what the basic underlying reality of the world is. What is matter composed of? Is all matter made of the same substance? Thales of Miletus (620–546 BCE) suggested that the fundamental basis for all material was water. Thales envisioned the Earth as a flat disk floating (resting) on water. This notion based on nature marked a shift away from divine explanations for celestial phenomena and toward scientific reasoning.
A student of Thales, Anaximander, revised these ideas and suggested that all matter in the world originates not from a formed substance like water but rather from apeiron, a boundless entity that pervades all of space. This led to the suggestion that a “plurality” of worlds could exist, with Earth perhaps being just one stage in a never-ending chain of worlds. About a century later, a school of thought called atomism emerged.
The Atomists
According to the atomists, among whom Democritus, Lucretius, and Epicurus were prominent, matter can be subdivided only to a certain point, at which only atoms (which cannot be cut, or are “indivisible”) remain. The world is made up of atoms moving in an infinite void. Atoms differed from each other only in size and shape, and different substances with their distinct qualities were made up of different shapes, arrangements, and positions of atoms. Atoms were in continuous motion in the infinite void and constantly collided (“swerved”) with each other. During these collisions they could rebound or stick together because of hooks and barbs on their surfaces. Thus, if life as we know it emerged this way, then the notion of life elsewhere in the universe forming this same way was within the realm of possibility. Thus, atomists also believed in a plurality of words — or many worlds. There was even speculation at this time, notably by Anaxagoras, that the Moon was rocky and reflected sunlight and was possibly inhabited by “lunarians”. For holding the belief that the Moon and Sun were not gods, Anaxagoras was sentenced to death for impiety.
Democritus gave some examples of how the atomic hypothesis could account for qualities such as color and taste (e.g., sharp tastes are caused by sharp atoms), but on the whole atomism, like other contemporary global theories, remained a general theory. It was criticized by Aristotle for some of its logical inconsistencies. For example, if atoms have different shapes, then they have parts, and this means that they are mathematically divisible; if they have different sizes, then among the infinity of their number there must be atoms as big as the world.
The Aristotelians
The Aristotelian description of nature was influenced by Pythagorean idealism. Plato suggested a mathematically perfect cosmos, in which the four fundamental elements – earth, fire, water, and air – are associated with regular solids. For example, fire is associated with a twelve-sided shape called a dodecahedron. Aristotle refined this cosmology and added the “aether”, or quintessence, to account for the void present in the atomist view and thus rendering the cosmos finite. In Aristotle’s view, all matter is at its natural state when at rest, and all objects will naturally fall back to Earth when displaced. Earth is naturally at the center in this system, in line with a geocentric viewpoint. This geocentric cosmology is counter to the plurality of worlds advocated by the atomists.
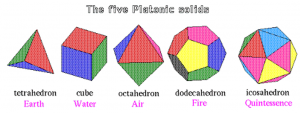
The last great astronomer of the Roman era was Claudius Ptolemy who flourished in Alexandria in about the year 140 AD. Ptolemy’s most important contribution was a geometric representation of the solar system that predicted the positions of the planets for any desired date and time. Ptolemy supplemented observational data collected by Hipparchus with new observations of his own and produced a cosmological model that endured more than a thousand years, until the time of Copernicus.
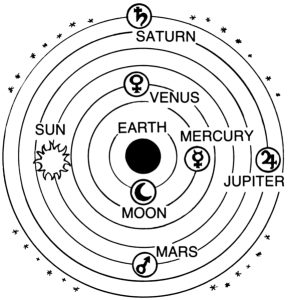
To fully explain the motions of bodies in the solar system, particularly the observation that Mars appears to change directions on the sky from time to time (this is known as apparent retrograde motion), some seemingly complicated behavior of the known planets at that time needed to be explained. A picture showing the apparent retrograde motion of Mars is shown in Figure 3. This time lapse sequence shows Mars on the sky over a period of about 8 months and shows that Mars appears to change directions and then change back as it moves on the sky. Trying to explain this unusual motion was a challenge in a geocentric model.
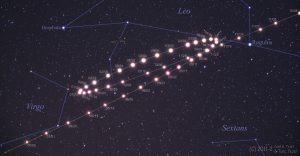
Because the Greeks believed that celestial motions had to be circles (Pythagoras, Plato), Ptolemy had to construct his model using circles alone. To do it, he needed dozens of circles, some moving around other circles, in a complex structure that makes a modern viewer dizzy (Figure 4). In order to match the observed motions of the planets, Ptolemy had to center the deferent circles, not on Earth, but at points some distance from Earth. In addition, he introduced uniform circular motion around yet another axis, called the equant point. All of these considerably complicated his scheme. The principle of Occam’s Razor states that given a number of different explanations, the simplest explanation is likely the correct one. Applying Occam’s Razor to the Ptolemaic model, the simplest explanation comes from a heliocentric model, which puts the Sun at the center (the Greek root helio means sun). However, the lack of evidence for a heliocentric model pushed the heliocentric model to the back burner until the Copernican Revolution.
The geocentric model of Ptolemy, with some modifications, was eventually accepted as authoritative in the Muslim world and (later) in Chr
istian Europe. The Aristotelian notions of purpose and order also fit the Christian mindset much better.
Concept Check
- The ancient Greeks ultimately adopted a geocentric model of the cosmos. If you could travel back in time and show the Greeks one modern observation to convince them that the heliocentric model is correct, what would the observation be?
- How would you explain this observation? Explain the meaning of the observation and how it supports a heliocentric model.
- Is this observation verifiable*? Explain why or why not you think so.
*In other words, could a scientist go out and take a measurement of that same observation today? For example, in assessing UFO claims, a person’s testimonial that they were abducted is not considered verifiable. However, a radio signal received at an observatory, perhaps from an alien civilization, could be verified by astronomers at other observatories.
The Middle Ages
Astronomy made no major advances in strife-torn medieval Europe. The birth and expansion of Islam after the seventh century led to a flowering of Arabic and Jewish cultures that preserved, translated, and added to many of the astronomical ideas of the Greeks. Many of the names of the brightest stars, for example, are today taken from the Arabic, as are such astronomical terms as “zenith.”
As European culture began to emerge from its long, dark age, trading with Arab countries led to a rediscovery of ancient texts such as Ptolemy’s Almagest and to a reawakening of interest in astronomical questions. This time of rebirth (in French, “renaissance”) in astronomy was embodied in the work of Copernicus.
The Renaissance and the Copernican Revolution
Copernicus
One of the most important events of the Renaissance was the displacement of Earth from the center of the universe, an intellectual revolution initiated by a Polish cleric in the sixteenth century. Nicolaus Copernicus was born in Toruń, Poland in 1473. His training was in law and medicine, but his main interests were astronomy and mathematics. His great contribution to science was a critical reappraisal of the existing theories of planetary motion and the development of a new Sun-centered, or heliocentric, model of the solar system.
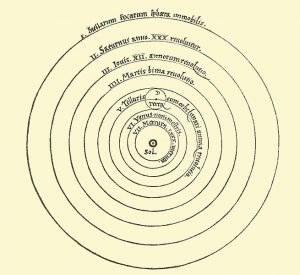
Copernicus concluded that all the planets circle the Sun and the Moon orbits Earth. Copernicus described his ideas in detail in his book De Revolutionibus Orbium Coelestium (On the Revolution of Celestial Orbs), published in 1543, the year of his death. The Copernican heliocentric model still adhered to the Platonic ideal of perfect circular motion at a constant speed, so the predictions made by the model did not perfectly match observations. This changed when Johannes Kepler, a German astronomer who was a contemporary of Galileo, empirically showed the planets move around the Sun in the shape of an ellipse. (Kepler’s Three Laws of Planetary Motion are discussed in detail in the Laws of Motion and Gravity chapter.)
Importantly, the heliocentric model provided a natural and simple explanation for the apparent retrograde motion of planets on the sky (Fig. 3 above). Whereas in the geocentric model a complex system with loops within loops was needed to explain apparent retrograde motion, it is a natural consequence of orbital motion in the heliocentric model. Figure 6 below shows this visually, which shows the situation for Earth and a planet that is further from the Sun, such as Mars or Jupiter. Earth is orbiting closer to the Sun than Mars and is moving faster around the Sun in its orbit than Mars does. As Earth and Mars orbit the Sun in in the same direction, Earth overtakes the planet periodically, like a faster race car on the inside track. From the perspective of the driver of the faster car, a slower car on the outside track will appear to move backwards when it is passed. The path of the planet among the stars is illustrated in the star field on the right side of Figure 6. The apparent motion backwards in the path Mars takes across the sky can be explained entirely due to our perspective on the Earth
.
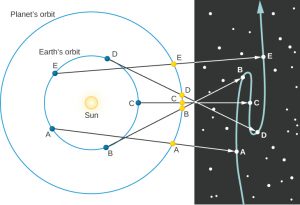
In the 16th century, the Danish astronomer Tycho Brahe collected very precise data of planetary positions, especially Mars, for twenty years at his observatory Uraniborg (the name refers to Urania, the Greek mythological goddess of Astronomy). Tycho hired Kepler to analyze this data, and Kepler eventually showed that the planets orbit the Sun in the shape of an ellipse, rather than a perfect circle. The entertaining video clip below summarizes Tycho’s work and life.
Galileo’s Observations
Two major milestones in Astrobiology happened around the same time in the 17th century: the invention of the telescope and the microscope — both are indispensable tools for this subject. It is not certain who first conceived of the idea of combining two or more pieces of glass to produce an instrument that enlarged images of distant objects, making them appear nearer. The first such “spyglasses” (now called telescopes) that attracted much notice were made in 1608 by the Dutch a lens maker. Galileo heard of the discovery and, without ever having seen an assembled telescope, constructed one of his own in 1609. The exact origin of the microscope is unknown but it likely emerged from the same setting in Denmark that saw the telescope emerge. The British physicist Robert Hooke coined the term “cells” after observing chambers inside of a piece of cork (cork is made of dead plant tissue) in 1665. By the late seventeenth century, the Dutch biologist Antonie van Leeuwenhoek had made pioneering strides in microbiology, particularly in the magnification of lenses, and discovered single-celled organisms (“animalcules”).
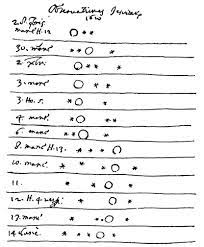
Galileo revolutionized astronomy by pointing his telescope to the heavens in 1609. He made some key discoveries and he published these results in his book Sidereus Nuncius (The Starry Messenger) in 1610. In addition to other findings, Galileo observed that the planet Venus goes through a full cycle of phases (just like the Moon does) as seen from the Earth, the Moon has “chains of mountains” and Jupiter has four moons that orbit around it. The observation of Venus’ phases was direct proof that Venus orbits the Sun, not the Earth, and showed that the geocentric model of the world was wrong. The observation that the Moon has mountains means that it is imperfect and therefore violates the Platonic ideal of mathematical perfection in the cosmos, further closing the door on the notion of geocentrism.
The observation that Jupiter had its own system of moons (see Galileo’s sketch on Figure 7) brings back ideas of a plurality of worlds. Earth is a planet with life and it has a moon orbiting around it. Since other planets also have their own moons, perhaps it is natural to assume that some of these planets also have life.
Johannes Kepler was aware of Galileo’s observations and interpreted this evidence as support for the idea that there could be other life in our solar system on both the Moon and Jupiter. Kepler wrote in 1610 in his letter Dissertatio cum Nuncio Sidereo (Conversation with the Sidereal Messenger) that “we deduce with the highest degree of probability that Jupiter is inhabited.” Galileo, however, did not think that there were worlds beyond our solar system, other than in a purely metaphysical sense.
The Dutch physicist Christiaan Huygens (1629-1695), who was the first person to observe the rings of Saturn and also discovered Saturn’s moon Titan, also advocated for other life in the universe. Although he did not think there was life on the Moon, since it doesn’t have an atmosphere, he imagined life on other planets in our solar system and even on planets around other stars. He speculated how plants and animals could differ on other worlds, depending on their gravity or thickness of their atmosphere. Huygens summarized these ideas in his book Cosmotheoros (New Conjectures Concerning the Planetary Worlds, Their Inhabitants and Productions), published in 1698 intentionally after his death (to avoid censure).
Religion and Science
The Inquisition was active during this time, and any persons denying the teachings of the Catholic faith were open to being accused a heretic. The Dominican friar Giordano Bruno (1548–1600) believed in an infinite cosmos composed of innumerable worlds and planets, an idea clearly at direct odds with the Catholic Church. Bruno’s cosmology consisted of a plurality of inhabited worlds, somewhat akin to the modern idea of parallel universes.
There are countless suns and countless earths all rotating round their suns in exactly the same way as the planets of our system. We see only the suns because they are the largest bodies and are luminous, but their planets remain invisible to us because they are smaller and non-luminous. . . . The unnumbered worlds in the universe are all similar in form and rank and subject to the same forces and the same laws.—Giordano Bruno in On the Infinite Universe and Worlds (1584)
Bruno was tried and accused of heresy and accordingly burned at the stake in the year 1600, a decade before the telescope would show that the cosmos was unimaginably vast.
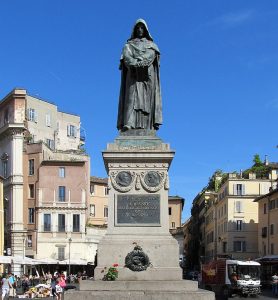
In this environment, there was little motivation to carry out observations or experiments to distinguish between competing cosmological theories (or anything else). It should not surprise us, therefore, that the heliocentric idea was debated for more than half a century without any tests being applied to determine its validity. (In fact, in the North American colonies, the older geocentric system was still taught at Harvard University in the first years after it was founded in 1636.)
Sometime in the 1590s, Galileo adopted the Copernican hypothesis of a heliocentric solar system. In Roman Catholic Italy, this was not a popular philosophy, for Church authorities still upheld the ideas of Aristotle and Ptolemy, and they had powerful political and economic reasons for insisting that Earth was the center of creation. Galileo not only challenged this thinking but also had the audacity to write in Italian rather than scholarly Latin, and to lecture publicly on those topics. For him, there was no contradiction between the authority of the Church in matters of religion and morality, and the authority of nature (revealed by experiments) in matters of science. It was primarily because of Galileo and his “dangerous” opinions that, in 1616, the Church issued a prohibition decree stating that the Copernican doctrine was “false and absurd” and not to be held or defended.
After Galileo’s work, it became increasingly difficult to deny the heliocentric view, and Earth was slowly dethroned from its central position in the universe and given its place as one of the planets attending the Sun. Initially, however, Galileo met with a great deal of opposition. The Roman Catholic Church, still reeling from the Protestant Reformation, was looking to assert its authority and chose to make an example of Galileo. He had to appear before the Inquisition to answer charges that his work was heretical, and he was ultimately condemned to house arrest. His books were on the Church’s forbidden list until 1836, although in countries where the Roman Catholic Church held less sway, they were widely read and discussed. Not until 1992 did the Catholic Church admit publicly that it had erred in the matter of censoring Galileo’s ideas.
The new ideas of Copernicus and Galileo began a revolution in our conception of the cosmos. It eventually became evident that the universe is a vast place and that Earth’s role in it is relatively unimportant. The idea that Earth moves around the Sun like the other planets raised the possibility that they might be worlds themselves, perhaps even supporting life. As Earth was demoted from its position at the center of the universe, so, too, was humanity. The universe, despite what we may wish, does not revolve around us.
Nineteenth and Twentieth Century
Ideas about other life in the universe continued to be debated into the 19th century. In 1853, the British philosopher William Whewell published Of the Plurality of Worlds, in which he argued against extraterrestrial life because he felt other planets in the solar system did not have the right conditions for life to survive.
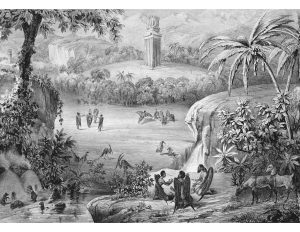
Around this same time, in 1835, the so-called Great Moon Hoax happened. The New York Sun, a then fledgling new paper, decided to print a series of stories reporting that there was life on the Moon. The articles provide details of the creatures that lived on the Moon, which included unicorns and lunar bats and quoted the astronomer John Herschel on these findings. Herschel, the son of the eminent William Herschel who had discovered infrared light, had just set up an observatory in South Africa and there was no reason to doubt an article that mentioned him. However, the articles were meant to be satire and were in fact not true!
Of course, that was probably not the first hoax claiming evidence for extraterrestrial life and not the last. In 1938, on the eve of Halloween, Orson Welles broadcast a dramatic rendition of the 1897 novel War of the Worlds by H.G. Wells. Although Orson Welles did not intend this to be interpreted as real, some listeners tuned in and believed that the Earth, specifically New Jersey, was under attack by Martians. Today, elaborate hoaxes claiming evidence of extraterrestrial life are fairly commonplace. A video was circulated in the 1990s that claimed to be evidence of an alien autopsy that happened at Area 51 in Nevada. Crop circles also appeared and these often complex structures were claimed to be the work of extraterrestrials. However, both cases were hoaxes.
Later into the 20th century, there was still belief that Mars was harboring intelligent life — some of this coming from claims made by the astronomer Percival Lowell who reported that there were man-made canals used for agriculture on Mars — but this generally was laid to rest after the Mariner missions sent back the first images of Mars in 1965.
Exercises and Activities
Summary Questions
- How do the views of what the universe is made of differ for the Atomists and the Aristoteleans?
- Describe Ptolemy’s geocentric model. Why was it so complex?
- Explain Occam’s Razor and how it applies to the Ptolemaic model.
- How did a heliocentric model of the cosmos come to be accepted during the Copernican Revolution?
- Describe and sketch a geocentric model, including the Earth, Sun, Moon and planets in our solar system.
- Describe and sketch a heliocentric model, including the Earth, Sun, Moon and planets in our solar system.
- Explain the apparent retrograde motion of Mars using the heliocentric model. It may be helpful to make a sketch or video.
- What did Galileo’s observe with his telescope in 1610? What is the significance of each of the five observations?
- Discuss why Galileo was accused of heresy by the Church in the 17th century.
Material Remixed From:
References
- https://www.daviddarling.info/encyclopedia/K/KeplerJ.html
- Wilkinson, D. (2013). ‘Speculating about a Plurality of Worlds: The Historical Context of Science, Religion, and SETI’, chapter in Science, Religion, and the Search for Extraterrestrial Intelligence. OUP Oxford.
"Before Common Era". The year 1 BCE is 2000 years prior to the year 2000 CE. Years in BCE increase in the direction of the past.