16 Searching for Life In Our Solar System
The Earth is the only place in our solar system that we know has life. Earth has some specific characteristics that make it habitable, such as plate tectonics and a surface gravity that prevents the atmosphere from escaping. What about the other planets in our solar system? By looking at the properties of each planet, and the Moon, we can assess if life could potentially exist in these environments.
Learning Objectives
By the end of this chapter, you will be able to:
- Discuss why the Moon and Mercury are very unlikely places for any life
- Discuss the possibility of life in the clouds of Venus
- Describe the main properties of the jovian gas giants and any possibilities for life
- Discuss different ideas on panspermia
Mercury and the Moon
Mercury and the Moon look very similar in photos, as seen in Figure 1 – both have surfaces that are heavily cratered and do not appear to have any clouds. The number of craters on a world is related to how geologically active that world is — active volcanoes, for example, will “pave” over craters and create a smooth surface. Mercury and the Moon, thus, appear to be geologically inactive.
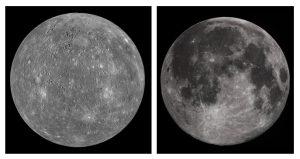
For most planets, their size and distance from the Sun can tell us most of what we need to estimate if they have the conditions to host life. Moons can also have the conditions for life but this depends on their distance from their host planet; we will see some examples of this with the jovian moons Europa and Enceladus. As noted in the previous chapter, the size of a world determines how quickly it will lose heat that is inside of it; the rate of heat loss depends inversely on the radius of the world. Mercury has a radius that’s only 38% the size of the Earth’s radius, and the Moon’s radius os 27% of the Earth’s, so both of these worlds lose their heat more quickly than larger terrestrial planets like the Earth and Venus.
For a world to be geologically active, there needs to be an internal heat source. Any heat that is left inside Mercury or the Moon is not enough to drive geological activity today, so we say that the Moon and Mercury are geologically dead. While it is not a strict requirement that a world be geologically active to harbor life (as far as we know), it has been extraordinarily beneficial for life on Earth, as plate tectonics and volcanism help to keep the Earth’s climate stable.
The Earth’s atmosphere is crucial to life on the surface of our planet. The surface gravity of the Moon is just one-sixth that of Earth and it is too weak to hold on to an atmosphere. Without a protective atmosphere to both shield and store energy from the Sun, the surface of the Moon experiences huge temperature swings. Without a global magnetic field, the Moon’s surface is bombarded by charged particles from the solar wind. The small traces of water that have been detected on the Moon are frozen as ice at the polar regions or in permanently shaded craters.
Like the Moon, Mercury does not have a significant atmosphere. However, Mercury a weak magnetic field has been detected on the innermost planet in our solar system, and this could help protect the surface from some of the pummeling by the solar wind. Because Mercury spins slowly, the days are very long on Mercury: one day on Mercury is the equivalent of 176 days on Earth. This leads to huge swings in Mercury’s temperature — temperatures reach a sizzling 430 °C (800 °F) on the sunlit side and drop to -170 °C (-274 °F) on the shaded side; water ice has been found in craters at Mercury’s north pole. Such a wide range in temperatures does not seem conducive for any type of life.
Since all life on Earth requires water, it makes sense to also assess if liquid water might exist on the surface of a world. A phase diagram shows the pressure (P) and temperature (T) ranges for the liquid-gas, solid-liquid, and solid-gas phase transitions (sometimes these diagrams are called P-T diagrams). Figure 2 below shows the phase diagram for water. The surface pressure for the Earth is shown for reference as the red line at $\sim$ 100 kPa (or 1 atm), with the freezing and boiling points of water indicated at 0 °C and 100 °C.

The Moon and Mercury have no atmospheres and therefore they have extremely low surface pressures. Water ice can exist in the coldest regions on the Moon and Mercury but can not make the transition to liquid water — the solid ice will go directly to gas (that is, it sublimates) at temperatures higher than the solid-gas transition point.
Concept Check: Phases of water
The surface pressure on Mars today ranges from 30 Pa on the heights of Olympus Mons to 1,115 Pa in the deep depression of Hellas Planetia. The average surface pressure on Mars is about 650 Pa. Can liquid water exist on the surface of Mars? If so, what temperature is needed?
Venus
Venus is sometimes referred to as Earth’s twin because the two planets have similar sizes and densities. From a great distance, Venus might be mistaken for a habitable world, and in the very distant past, it is possible that oceans of water and life did exist on Venus. Pictures of the Venusian surface show clouds that with a pinkish hue, and Venus shows evidence of recent volcanism[1]. But the runaway greenhouse that occurred on Venus has made the planet inhospitable for life today. The loss of water and the build up of a thick carbon dioxide atmosphere has led to a crushing atmospheric pressure, 90 times stronger than experienced on Earth’s surface.
This dense atmosphere leads to a bone-dry surface with a fairly uniform temperature of 850 °F on Venus. The Venusian surface is too hot for any (known) life to survive on. When the Venera 13 spacecraft touched down on Venus in 1982, it captured data for about 2 hours before the electronics were fried and the pressure crushed it to death (Figure 3).

Another big difference between Earth and Venus is the rotation rates – how fast the planets spin: a day on Venus lasts 243 Earth days. As evidenced by volcanic mountains Venus does have a molten layer to its core but the slow rotation rate impedes the formation of a global magnetic field. Without a magnetosphere for protection from the solar wind, the top layers of Venus’ atmosphere are slowly being stripped away.
Life in the Clouds of Venus?
Before completely dismissing the possibility of life on Venus, let us look at its atmosphere in more detail. The temperature of the Venusian atmosphere varies with altitude, as seen in Figure 4. In the upper troposphere, between 30 and 60 kilometers above the surface, a thick cloud layer is composed primarily of sulfuric acid droplets. Sulfuric acid (H2SO4) is formed from the chemical combination of sulfur dioxide (SO2) and water (H2O). Sulfur dioxide is added to the atmosphere via volcanic eruptions on Venus’ surface. In the atmosphere of Earth, SO2 is one of the primary gases emitted by volcanoes, but it is quickly diluted and washed out by rainfall. The temperature in parts of this cloudy region are between 273-373 K, making this a layer with the conditions for habitability. Any bacteria that could thrive in this zone would take in sulfur as a nutrient. On Earth, bacteria that survive on sulfur are found in rocks that date back to around 3 billion years, prior to the Great Oxidation Event.

In an article published in the journal Nature in 1967, “Life in the Clouds of Venus?”, co-authors Harold Morowitz and Carl Sagan note that:
“While the surface conditions of Venus make the hypothesis of life there implausible, the clouds of Venus are a different story altogether. As was pointed out some years ago, water, carbon dioxide and sunlight—the prerequisites for photosynthesis—are plentiful in the vicinity of the clouds. Since then, good additional evidence has been provided that the clouds are composed of ice crystals at their tops, and it seems likely that there are water droplets toward their bottoms. Independent evidence for water vapour also exists.”
No life has yet been detected in Venus’ clouds, although there was cautious excitement in 2020 when the detection of phosphine (PH3) in the atmosphere of Venus was announced. The “Extraordinary Claims” box below describes the arc of this announcement and subsequent denouncement.
Extraordinary Claims: Life in the clouds of Venus
In September 2020, an article was published in the journal Nature titled “Phosphine gas in the cloud decks of Venus.” The article immediately gained widespread attention, as the molecule phosphine (PH3) is produced on Earth by bacteria. In other words, phosphine could be a potential biosignature, or sign of life, on Venus. The authors note in the paper’s abstract that “PH3 could originate from unknown photochemistry or geochemistry, or, by analogy with biological production of PH3 on Earth, from the presence of life.”
The claimed detection of PH3 was based on spectroscopic data taken using two different telescopes. Very small amounts of phosphine are found in Earth’s atmosphere, primarily from microbial life living in very low-oxygen environments (phosphine is toxic to humans). Phosphine can also be produced during the production of methamphetamine, which is also a man-made, or biological, source. Because of the potentially revolutionary impact of this discovery if true — this would be the first detection of any life elsewhere in the cosmos — other scientists were eager to verify the detection and rule out any ways that PH3 could be produced naturally on Venus.
In February 2021, a study was published by astrobiologists at the University of Washington that used atmospheric models to reproduce the PH3 detection but found instead that sulfur dioxide (SO2) was a far more likely explanation than phosphine. NASA’s SOFIA mission also searched for the PH3 line but did not find any evidence for it. Others have speculated on ways that PH3 could be produced abiotically (meaning not by life) on Venus, including by phosphorous from a volcanic eruption interacting with sulfuric acid in Venus’ clouds.
While the case is not totally closed on the possibility of PH3-based life in the clouds of Venus, other studies would need to corroborate the evidence for this claim to be accepted.
References
Bandari, A. (2022, November 29). No Phosphine on Venus, According to SOFIA – SOFIA: Stratospheric Observatory for Infrared Astronomy. https://blogs.nasa.gov/sofia/2022/11/29/no-phosphine-on-venus-according-to-sofia/
Greaves, J. S. et al. (2021). Phosphine gas in the cloud decks of Venus. Nature Astronomy, 5(7), 655-664.
Lincowski, A. P. et al. (2021). Claimed detection of PH3 in the clouds of Venus is consistent with mesospheric SO2. The Astrophysical Journal Letters, 908(2), L44.
Witze, A. (2021). Life on Venus claim faces strongest challenge yet. Nature, 590(7844), 19–20. https://doi.org/10.1038/d41586-021-00249-y
The Gas Giant Planets
The jovian planets are gaseous (Jupiter and Saturn) or a slushy ice mix (Uranus and Neptune) and do not have solid surfaces; thus, any life on these giant planets seems virtually impossible. These planets orbit far from the Sun and do not intercept enough solar energy for life as we know it to thrive (the upper atmosphere of Jupiter, for example, has an average temperature of -110 °C[2], much colder than the lowest known temperature for any psychrophiles), especially without surface chemistry to spark life and sustain evolution. That said, we can still make a critical assessment similar to that made for Venus to see if there are layers in the atmospheres of the gas giants could potentially have conditions that could harbor life.
The Jovian Atmospheres and Clouds
Looking at the atmospheres of the jovian giants in Figure 5, we see that all of the crucial elements for life — CHNOPS — are all seen in various molecules. For Jupiter and Saturn, there is a temperate region which has water (H2O) droplets. There are also organic compounds, such as methane, that may provide a source of nutrients, and there is still leftover heat from formation that keeps the atmosphere warm, at least close to the surface.

The possible layer of water clouds in Jupiter’s clouds present an intriguing environment for possible life. In the 1970s, Carl Sagan and E. E. Salpeter imagined an entire ecosystem existing within this layer, with organic life forming from methane and electricity. Once formed, these organisms could exist on energy from the Sun and nutrients in the atmosphere (such organisms are called phototrophs). Evolution could act on these organisms and larger, buoyant life forms that can adapt to different pressure levels in the atmosphere could exist and compete for resources. The downside of this scenario is that the atmosphere of Jupiter is very turbulent, with storms raging for centuries with winds that may be too high to sustain such life.
Carl Sagan discusses the origin of life.
- How do experiements to synthesize biomolecules in terrestrial labs inform our understanding of the origin of life elsewhere?
- What might life on Jupiter look like? What are enviromental limitations for putative organisms on Jupiter?
Video source: @yeoldebrian
Panspermia
In our discussions of the possibility of life elsewhere in our solar system, we have not specifically addressed the question of whether life on Earth could have originated somewhere else and ended up on our planet. This possibility is not unfeasible: we have evidence that other worlds in our solar system, like Venus and Mars, were once habitable places long ago, and perhaps back then a microbe could have hitched a ride to Earth on a meteor. Could life have come from outside our solar system? This seems less likely, merely based on the extreme distances that the life would have to travel and survive before getting to Earth, but still worth considering. A more speculative idea is that an extraterrestrial civilization could have brought life here intentionally.
The video clip below reviews some of the ideas related to panspermia.
- What are some adaptations of life on Earth that might allow organisms to survive in space?
- What transport mechanisms might exist for tiny organisms to move to other planets?
- How long can extremeophile organisms remain dormant while traveling through space?
Video credit: @bbcideas and The Open University
Interplanetary
If life developed on Earth so quickly, and other terrestrial planets (Venus and Mars) had similarly habitable conditions, it is easy to imagine that life may have evolved independently on those planets around the same time it did on Earth. We have strong evidence, for example from stromatolites and microfossils, that life formed on Earth fairly rapidly (3.5 to 4 billion years ago), perhaps even during the Hadean eon when liquid water oceans first appeared.
If life did form independently on another planet in our solar system, then it could have travelled to Earth in its early stages. There were far more collisions in the early days of the solar system and this could have provided a vehicle for a rock with life to be blasted from one rocky planet and land on another. Of course, life surviving the impact poses challenges but this is nonetheless a plausible scenario. We have learned from extremophiles on Earth that microscopic life can be remarkably resilient. Another possibility is that small dust grains could be released during the impact and these could make their way to another planet (rather than surviving on the rock itself).
Comets, Asteroids, and Meteors
Comets, asteroids and meteors are leftover debris from the formation of the solar system and therefore provide a way of sampling the conditions in the very young solar system, before life emerged on Earth. Could life have formed on these relics? With the exception of the asteroid Ceres (more on that in a bit), these objects are far too small to have any heat inside of them (remember that small worlds lose their heat more rapidly than larger ones). There is therefore no heat to drive geological activity and there are no protective magnetic fields. Adding to this the frigid temperatures that prohibit liquid water from existing, we can rule out any life on these objects.
However, the building blocks for life, amino acids, have been found on the surface of all of these types of objects. For example, the Rosetta mission detected glycine on the comet 67/P. Analyses of the Murchison meteorite, which landed on Earth in Australia in 1969, have shown that it contains more than 90 amino acids, many not found on Earth, including some of the 20 amino acids that are used by all life on Earth. Organic materials have also been found on the asteroid Ryugu, from which a sample was collected by the Japanese Aerospace Exploration Agency’s Hayabusa2 mission and successfully brought back to Earth for analysis. (The OSIRIS-REX mission dropped off samples from asteroid Bennu on Earth in September of 2023.) While life does not exist on these bodies, they could have brought the ingredients for life to Earth or others places in the solar system via a collision. This is strictly not panspermia, since life itself was not delivered, and is sometimes called pseudo-panspermia to make this distinction clear.
Life on Ceres?
The asteroid Ceres, orbiting in the asteroid belt between Mars and Jupiter, is large enough to also be classified as a dwarf planet. Ceres was explored in detail by NASA’s Dawn mission from 2015-2018. Dawn detected organic molecules on Ceres’ surface, some of them the building blocks for amino acids used for life on Earth, although it is not clear if these organics formed on Ceres or were brought there from other bodies. Dawn studied bright white spots on Ceres (Figure 6) that are associated primarily with the central peaks of large craters, such as the Occator crater, and found that the light-colored mineral is primarily salt, released from the interior[3]. After repeated close flybys, data from the Dawn spacecraft indicated that Ceres has (or has had) briny water beneath its surface that can be brought up through volcanoes. The most dramatic is the 4 kilometer tall ice volcano called Ahuna Mons. This raises the possibility that Ceres is still geologically active today, and that it was once a habitable world.
The TEDx Talk below discusses some potential avenues for life coming to Earth via panspermia. After watching the video, consider the following discussion questions:
- In addition to tardigrades, what type of bacteria can survive harsh environments? What can these bacteria do that makes them particularly robust?
- Discuss the possibility of life originating in the Jezero crater on Mars.
- Which two missions are currently searching for signs of organic matter in asteroids and comets? How and where are they searching?
Video Credit: @TEDx
Interstellar Panspermia
The principle of life being brought to Earth from another solar system shares the same basic premise as interplanetary panspermia, but with much longer distances for the life to travel. The challenges are the same but on a much larger scale, as the life must survive a longer trip once it is ejected from another solar system. Thus, there is a much lower probability of life arriving from an interstellar rock or comet than from within our solar system. However, this possibility seems more pertinent now that two interstellar objects, 1I/Oumuamua and 2I/Borisov, have been detected in our solar system, where the designation “I” stands for Interstellar. Oumuamua is believed to be an asteroid and Borisov is likely a comet, so we have confirmation that objects from other star systems can reach our own. In addition to interstellar asteroids or comets, life could potentially be transferred via much smaller dust grains (on the order of microns, or 10-6 meters) that still can potentially provide protection from dangerous radiation on a journey between stars.
Directed Panspermia
There is of course another possibility, that life was planted on Earth by a technologically advanced extraterrestrial civilization. This idea may seem far-fetched but it has received serious consideration, notably from Francis Crick (who co-discovered the double-helix structure of DNA) and Leslie Orgel. In 1973, Crick and Orgel published a paper describing their idea of so-called “directed panspermia,” in which life on Earth was brought here as microbes on a spacecraft from another another planet that is occupied by advanced life[4]. One of their arguments in favor of the idea that Earth was “infected” with alien life is that humans could conceivably do that same thing; that is, we could send microbes out to seed another world with life. They call this premise the idea of “cosmic reversibility” — if we could do it, so could another civilization. One way to find evidence that we were infected in this way would be to search for signs of it in the genetic code. More recent ideas of directed panspermia suggest that the extraterrestrial civilization would use a comet to spread life, rather than a spaceship.
Key Concepts and Summary
Small bodies like the Moon and Mercury do not have sufficient surface gravity to hold on to an atmosphere and have also lost most of their internal heat. Venus is similar in size and mass to the Earth and may have had habitable conditions at one time. However, a runaway greenhouse effect on Venus produced a massive, crushing atmosphere and raised the surface temperature so that complex biochemistry would only be possible in the upper atmosphere. The gas giant planets, Jupiter and Saturn have massive atmospheres with no real solid surfaces and relatively small high-density cores. The search for life in the solar system is really a search for life as we know it on Earth. If similar biochemistry is found on other solar system bodies, this might support the theory of panspermia – the idea that life originated beyond our planet (or solar system) and was transported here.
Review Questions
Summary Questions
- Are Mercury and the Moon geologically active today?
- Why are Mercury and the Moon not likely to host any life?
- Why can’t life exist on the surface of Venus today?
- What are Venus cloud layers made of, and in which layer could life possibly exist?
- Discuss the reported detection of phosphine in Venus’ clouds and why this was of interest to Astrobiologists. As of today, has the detection been confirmed?
- What makes the surface and interiors of the jovian gas giants inhospitable for any life?
- How did Carl Sagan imagine life in the clouds of Jupiter? Do you think this scenario is likely? Explain why or why not.
- What is the main idea of panspermia?
- How could life travel between planets?
- Where else in our solar system have amino acids been found?
- Could life exist on the asteroid/dwarf planet Ceres? Explain.
- What additional challenges are posed when considering interstellar panspermia?
- What is the main idea of directed panspermia? Do you think directed panspermia is likely? Why or why not?
Exercises
- https://www.science.org/content/article/active-volcano-shows-venus-living-planet ↵
- https://science.nasa.gov/resource/solar-system-temperatures/ ↵
- Read more: https://www.nasa.gov/centers-and-facilities/jpl/mystery-solved-bright-areas-on-ceres-come-from-salty-water-below/ ↵
- Crick, F. H., & Orgel, L. E. (1973). Directed panspermia. Icarus, 19(3), 341-346. https://doi.org/10.1016/0019-1035(73)90110-3 ↵