8 Alternative Biochemistries: Speculations about Strange Possibilities
One can only imagine how the field of biology will change the moment extraterrestrial life is discovered for the first time. Will we see shocking similarities? Or will life elsewhere be so different that it is barely recognizable to us?
Learning Objectives
By the end of this chapter, you will be able to:
- Discuss the viability of silicon-based life
- Discuss why liquid water is a useful solvent for life
- Describe some possibilities for alternative nucleic acids
Alternatives to Carbon
While carbon is clearly favored for Earth-based organisms, other hypothetical biochemistries have been considered by biochemists. One of the most frequently imagined alternatives is silicon-based life. Silicon is similar to carbon in that it also has four valence electrons. However, these electrons are in silicon’s third electron shell while carbon’s valence electrons appear in its second electron shell. The additional shielding of valence electrons by two inner filled shells of electrons is enough to change the nature of silicon bonds. Because of the valence electrons of silicon are farther from the positively charged nucleus, silicon bonds are weaker than carbon bonds. While carbon can effectively form the complex long molecular chains necessary for life, it is rare to find more than three silicon atoms in a single molecule. Even when they do form, compounds with multiple silicon atom have bonds that are easily disrupted by water. The difference in valence shell energy also makes it harder for silicon atoms to form double or triple bonds. Therefore, silicon molecules seldom exhibit chirality.
Because the valence electrons of silicon are more weakly held to the atom, it is easier for oxygen to bond with silicon, but it is also much harder to break these bonds. Once oxygen has bonded with silicon, it takes a lot of energy to pull it away. Biological processes often require recycling elements into different organic compounds. The difficulty in breaking down silicon-oxygen molecules slows the reaction rates. The structure of silicon-oxygen bonds also renders many important organic molecules unfriendly for life. For instance, we exhale carbon dioxide, CO2, as part of the process that generates energy. The silicon counterpart of CO2, silicon dioxide, is a solid rather than a gas, making it much harder to dispose of than carbon dioxide. In general, silicon tends to form more solid, crystalline structures when compared to its carbon counterparts.
We should not assume that silicon will “never” be a good choice for biochemistry. However, the laws of chemistry seem to strongly favor carbon-based life, especially in temperature and pressure environments found on Earth. The next time your favorite science-fiction movie portrays silicon-based life, remember that it should expel a mouth full of silicon dioxide crystals with every breath.
Water as life’s solvent
Water is thought to be essential to life both because where there is life there is water and where there is water there is life. Water possess many unique chemical properties that make it conducive to both life and the organic reactions that govern it.
Water makes up the most of most living systems. The average organism is 70% or more water by weight. Recall that water, or H2O, consists of one oxygen molecule and two hydrogen molecules. As mentioned before, oxygen is particularly good at attracting electrons. Even within a molecule of water, the electrons preferentially spend time closer to the oxygen molecule than to the hydrogen atoms. Because electrons carry a negative charge, the result is that the oxygen molecule is slightly negative while the hydrogen atoms are left with a slightly positive residual charge.
The charge differential across the water molecules makes water polar and consequently useful for organic chemistry in several ways. The different charges help group nonpolar molecules together and create effects like self-assembling phospholipid structures. Proteins are also folded with the help of water when amino acids with nonpolar side chains are naturally folded inside more water-friendly side chains.
Because of its polarity, water has a strong bond to neighboring water molecules. Water molecules can form hydrogen bonds with one another. These bonds help water to remain liquid over a greater range of temperature. Water provides a safe, stable environment to nurture the complex reactions required by life.
The difference in charge also allows water to more easily pull compounds apart, making it easier for chemicals (like the salt crystal in Figure 2 below) to dissolve in water. This has earned water the moniker “the universal solvent.” Once in the water, chemicals are free to move around and interact with other chemicals.

A crystal of table salt (NaCl) dissolving in water. The table salt is easily dissolved into negatively charged chlorine ions (green) and the positively charged sodium ions (yellow) in water where the ions are stabilized through interacting with the differently charged ends of water. What section of the water molecule preferentially faces the sodium atom and which end preferentially faces the chlorine atom? Recall that it is opposite charges that attract.
When water freezes into ice, the positively-charged hydrogen atoms align with the negatively-charged oxygen atoms, forming an organized lattice. In this highly-organized configuration, the molecules in ice are more widely spaced than molecules in water, resulting in a lower density that allows ice to float on top of water. This property carries many benefits for biology as ice can therefore serve as a protective barrier for the water underneath. It is capable of blocking out potentially damaging radiation and cooling water to stabilize certain compounds.
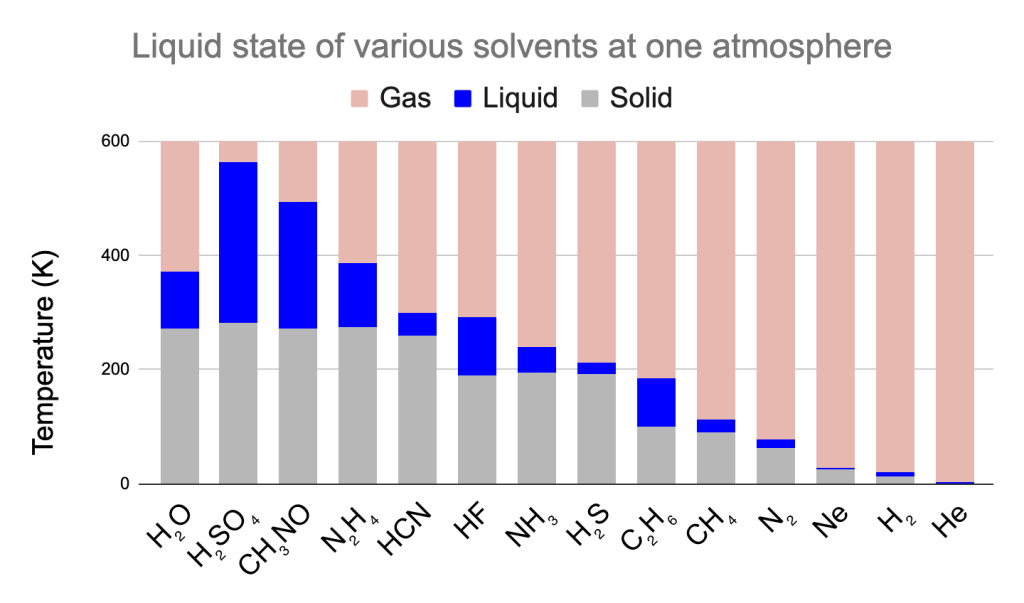
Could life outside the Earth be based on a different liquid solvent? There are a few considerations to make. If we assume that the liquid state is what is required for biochemistry, then we might consider the range of possible temperatures at which a particular chemical is liquid. This is dependent on the atmospheric pressure with the range increasing as the pressure increases. For convenience sake, the range of temperatures where various chemicals are liquid at standard atmospheric pressure (usually designated with the measurement “one atmosphere”) is given in Figure 3. The compounds which are liquid over the largest range of temperatures are constitued bypolar molecules similar to water and generally liquid at temperatures that exceed 200 K.
Scenarios where one of these other chemical compounds would be the dominant liquid on a planet’s surface are subject to the availability of the chemical to the exclusion of others. This is an important consideration as water as a chemical compound is observed to be rather common throughout the universe. The instances where water is not available to act as a solvent in our own solar system are largely due to either the conditions being such so that the material is either permanently frozen solid or is in a perpetual gaseous state sometimes escaping the gravitational pull of the world entirely. In most instances, this results in a dry world with no surface liquid present in any abundance. Other than the Earth, the one exception to this in our own solar system is Saturn’s moon, Titan, which has liquid methane (CH4) and ethane (C2H6) on its surface–this liquid being dominant mostly because the surface temperatures are so low.
Alternatively, subsurface liquids are known to exist in many worlds and it is likely that most of the liquid chemicals in our solar system are contained in such interior oceans. The higher interior pressures beneath solid surfaces provides for environments that are well-suited to preserving liquid state as long as there is some energy source to keep the interiors at high enough temperatures. In many moons, this energy source is derived from tidal stresses. In contrast to larger worlds, they are small enough to have lost the internal heat that was present at their formation and, unlike the Earth, far fewer radioactive elements are present, so some external source of heating is required to keep the interiors warm. The challenges of studying subsurface oceans are similar to the challenges we have on Earth in attempting to access the interior of our own planet compounded by the space travel necessary to reach these distant worlds. Thus, we have yet to directly access any of these subsurface oceans to confirm their composition, but evidence seems to point to many if not most of these subsurface oceans are water.
However, some worlds seem to have interior conditions that might not allow for liquid water. For example, there is evidence that Neptune’s largest moon, Triton, has a reservoir of liquid nitrogen beneath its surface. Such cryoliquids (chemicals that are liquid at very low temperatures) could exist in contexts where temperatures were too cold for liquid water. In many cases, these cryoliquid chemicals are made of nonpolar molecules. At these incredibly low temperatures, chemistry happens at far slower rates and there are some who question whether complex organic chemistry can happen at all, but such bizarre environments are very difficult for us to test carefully since even if we can afford to run experiments at these very cold temperatures, we tend to require human-timescales to run experiments.
Alternative Nucleic Acids
The RNA World Hypothesis sets forth an intriguing possibility that the first proto-life on Earth was derived primarily from RNA and only later did lipids, polypeptides, and DNA become crucial to biochemistry. However, are DNA and RNA the only possibilities for such nucleic acids?
One possibility that briefly excited some in the news media was the claimed discovery in 2010 of a bacteria called GFAJ-1 that appeared to be based on a nucleic acid that had an arsenic backbone rather than the phosophorus backbone of DNA and RNA. Like silicon and carbon, arsenic and phosphorus share similar chemistries. Unfortunately, this claim was disputed by most independent experts who evaluated the claim and a study in 2012 showed that the organism instead of using arsenic actually still relied on phosphorus and was simply arsenic resistant.
More generally, some scientists have speculated and even created entire Xeno Nucleic Acids (XNA) in laboratory environments which use different backbones, sugars, and base pairs. Such study is part of the larger endeavor of xenobiology which involves the creation of biologically active chemicals that are not found naturally. Of course, just because such xenobiological chemicals are not found in terrestrial habitats, this does not mean there are no instances of such in the vast universe of possibilities beyond our Earth.
Key Concepts and Summary
Every form of life on Earth uses carbon as the basic atom for biochemistry. We can imagine other types of chemistry for other environments and there has been some speculation about silicon chemistry as an alternative. Like carbon, silicon has four valence electrons, however this is in the third shell rather than the second shell and the silicon bonds are much more fragile than carbon. On Earth, all life requires water as a solvent but on other worlds, there could be non-water solvents. In addition, life elsewhere could use different amino acids and a different genetic code – all of this will serve to make life elsewhere more difficult for us to recognize.
Review Questions
Exercises
- Investigate XNA possibilities. Below is a list of different nucleic acids which are not used by terrestrial lifeforms. Choose one of them and discuss its chemical properties. Include a diagram in your discussion of how the chemical differs from that of DNA and RNA:
-
Peptide nucleic acids (PNAs)
-
Threose nucleic acid (TNA) or α-(L)-threofuranosyl-(3′-2′) nucleic acid
-
Glycol nucleic acid (GNA)
-
1,5-Anhydrohexitol nucleic acid (HNA)
-
Cyclohexene nucleic acid (CeNA)
-
Morpholino
-
Locked nucleic acids (LNA)
-
Fluoroarabino nucleic acid (FANA)
Each of these artificial nucleic acids differs from naturally occurring DNA or RNA by changes to the molecule’s backbone. -
Summary Questions
- Why was silicon-based life considered a possibility by some speculative thinkers? What makes it likely or unlikely to exist?
- What features of water allow it to be a useful solvent for life?
- Are there other possible liquid solvents for life? If so, how might they better or worse than water as a solvent?
- What parts of DNA might be modifiable to produce alternative nucleic acids that other forms of life might be able to use?