10 From Biochemistry to Biology
How did the first life emerge on Earth? At some point, abiogenesis — the transition from chemicals to living cells — occurred. Since there is no direct evidence of this first life, scientists must rely on experiments that try to recreate the conditions on the early Earth to see how easily the building blocks of life can assemble.
Learning Objectives
By the end of this chapter, you will be able to:
- Describe the Miller-Urey experiment and how it contributes to our understanding of how life formed on Earth
- Discuss the main ideas of the RNA World hypothesis for how life formed on Earth
- Discuss how synthetic biology informs the study of the emergence of life on Earth
- Describe how vesicles can form spontaneously from prebiotic chemistry
- Discuss how the first protocells formed and could plausibly replicate
The Building Blocks of Life
What were the conditions on the early Earth like? After the Earth formed and the period of heavy bombardment ended, chemical reactions occurred between simple molecules, leading to more complex molecular structures. How far can natural processes go toward the formation of the first biological cell? How can the non-living suddenly become alive? The study of prebiotic chemistry has yielded some important insight into these questions.
The Miller-Urey Experiment
Once biochemists understood some of the important chemistry in living organisms, a new field of synthetic biology was born. Biochemists tried to replicate the pathway for the evolution – or the complexification – of simple chemistry. Could chemicals on the early Earth provide a pathway to the formation of the first living cells?
In 1953, Stanley Miller and Harold Urey published the results of their now famous experiment: they spontaneously produced amino acids from simple elements under conditions that they believe emulated early Earth. Given the importance of amino acids and proteins for life, this experiment was viewed as an important step toward understanding the origin of life.

The experimental setup, depicted in Figure 1 above, consisted of two large flasks. One flask contained gases thought to be present in the primitive atmosphere (methane, ammonia, and hydrogen) and the other contained water, representing the ocean. Electrodes were inserted into the atmosphere flask to produce electrical sparks that would emulate lightning.
The ocean flask was heated, producing water vapor that traveled throughout the setup and interacted with gases in the atmosphere and simulated lightning. The enriched vapor then traveled through a condenser and cooled to a liquid state in a u-bend at the bottom of the setup where samples could be collected. Within a day of running the experiment, the liquid at the bottom of this u-bend had turned pink. The inaugural run of the experiment lasted for a week. From these results, Miller was able to identify five different amino acids that had been created from this simple set up of atmospheric gases, lightning, and water alone. As expected, the chirality of these amino acids included both left- and right-handed molecules, even though only left-handed amino acids are incorporated into biology as we know it today. Recent reanalysis of sealed vials have detected more than 20 different amino acids that were actually formed during that one-week experiment.
Since 1953, there have been many additional experiments that aim to synthesize the building blocks of life. Longer chains of amino acids and polypeptides have been synthesized. An impressive experiment was carried out by John Sutherland’s lab in 2009. Sutherland began with a compound called 2-amino-oxazole, and using phosphorus as a catalyst, he was able to synthesize some of the nucleotides used in RNA and DNA.
Want to know more: The Murchison Meteorite – amino acids in space
On September 28, 1969, two months after Apollo 11 landed on the moon, a huge meteorite soared through the sky and landed near Murchison, Victoria, in Australia. The Murchison Meteorite is one of the most valuable and well-studied meteorites. Importantly, the incoming meteorite was highly visible. It flew through the atmosphere as a fireball and caused a noticeable tremor when it landed. This allowed for rapid recovery of the meteorite, preventing terrestrial contamination. A piece of the Murchison meteorite is shown below.
In total, more than 100 kg (the average weight of an NFL linebacker) of the Murchison Meteorite was recovered over an area of 13 square kilometers. This meteorite contained more than 70 amino acids, many of which are not known to exist naturally on Earth. There is a slight over-abundance of left-handed amino acids, which suggests that there may be a cosmic origin to the dominance of left-handed amino acids that are used by life on Earth. Every amino acid discovered on the Murchison Meteorite has since been successively synthesized using a Miller-Urey-like setup with gases, water, and simulated lightning, demonstrating that these amino acids can spontaneously form under fairly simple conditions. The Murchison Meteorite is proof that amino acids can easily form in space. Amino acids: so easy to synthesize that a rock can do it!
Even more complex organic molecules have been detected in interstellar space, a testament to how easily complex, carbon-based structures can form and remain stable. Some of these molecules have carbon ring-structures called polycyclic aromatic hydrocarbons and are similar to the organic molecules found in biological organisms. This confirms that carbon is a good base for forming complex molecules structures, including amino acids. While this gives a hint that carbon-based life may also exist on other planets, there are still many steps we don’t yet understand about how to go from prebiotic chemistry to life.
Abiogenesis
Keep in mind that just because we discover processes that can create the chemicals we have identified as vital to living things does not answer the question of how life emerges. The transition from such chemicals to lifeforms and biotic systems is a process generally called abiogenesis. Many mysteries remain in the study of this question.
Once amino acids are formed, they can link together through peptide bonds to form chains (see the section on “amino acids to proteins” for a review). These polypeptide chains can fold into proteins, which perform functions essential for life. Thus, we see a natural increase in the chemical complexity of simple molecules, leading from monomers to polymers. Just as amino acids polymerize to form proteins, another crucial step in the origin of life involves the polymerization of nucleotides to form RNA (see “nucleic acids to genes“). Let’s explore how the RNA world hypothesis provides insights into the early development of self-replicating systems and the foundation of genetic information.
RNA World
Proteins are needed to catalyze chemical reactions critical to the survival of cells. But, it is difficult to imagine how proteins could have been the precursor to living cells because DNA is required to manufacture proteins, presenting a “chicken-and-egg” problem. A breakthrough came when Sidney Altman and Thomas Cech discovered a class of RNA molecules called ribozymes that could catalyze their own replication. Ribozymes show that RNA, which can encode genetic information, can also act as an enzyme. This discovery was awarded the Nobel prize in Chemistry in 1989 and supported a hypothesis called the Early RNA world, where ancient life used RNA for storing genetic information and catalyzing chemical reactions. This hypothesis had been suggested in the 1960’s by Carl Woese, Frances Crick and Leslie Orgel.
After watching this video, answer the following questions:
- What are three reasons why RNA is thought to have given rise to the first life?
- Explain how mutations contribute to the evolution of RNA strands.
- How can a single strand of RNA become a ribozyme? Draw a sketch of this process.
Video source: @StatedClearly
According to this hypothesis, the instability of RNA promoted mutations and natural selection eventually evolved a more stable, double-stranded DNA molecule as ribozymes were phased out. A fascinating “smoking gun” for this hypothesis is the fact that the ribosome, which assembles proteins in cells today, is a ribozyme! While the current day ribosome incorporates some proteins, none of the proteins are anywhere near the active site where chemical reactions take place. They appear to exist largely for structural support for the ribosome.
While the capabilities of RNA seem to make it the perfect candidate to explain the origin of life, RNA is a far more complicated structure and not as easy to make as amino acids. Miller-Urey experiments have been capable of synthesizing a series of smaller, very reactive molecules. When enough of these molecules are made, detectable amounts of purine and pyrimidine bases, which are essential components of nucleotides needed for RNA, can be detected. Components of nucleotides have also been discovered on meteorites like the Murchison Meteorite. However, no complete extraterrestrial nucleotides or nucleic acid chains have been discovered yet.
A breakthrough came in 2009 from British chemist John Sutherland along with Matthew Powner and Beatrice Gerland (read more here about their experiment and results). Sutherland’s group determined a chemically efficient pathway for nucleotides to form that is plausible in a prebiotic environment. Rather than form each component of the nucleotide individually, which would require separate and unlikely chemical environments, they proposed a method that formed and attached a purine and a ribose sugar in the same reaction. Phosphate is used to help catalyze the reaction and incorporated into the nucleotide later. This work showed that the building blocks of RNA could form naturally on the early Earth.
Knowing that RNA nucleotides can form under prebiotic conditions, how can these nucleotides assemble into self-replicating RNA molecules? In 2009, Drs. Tracey Lincoln and Gerald Joyce created an RNA enzyme that was capable of self-sustained replication indefinitely (read their paper in Science).
Dr. Gerald Joyce discusses research in his lab on the self-replication of RNA.
After watching the video, consider the following discussion questions:
- Why are scientists interested in creating self-replicating systems?
- According to the timeline of events on Earth that Dr. Joyce shows, when did abiogenesis most likely occur on Earth?
- What is the “Replicator” that Dr. Joyce refers to?
- What biological process do these synthetic systems mimic? (cell division)
- Summarize the first method that Dr. Joyce discusses on how to build a replicating system using just prebiotic chemistry.
Video source: @MoleCluesTV
Understanding how nucleotides can assemble into self-replicating RNA molecules explains part of the puzzle of how biological life began. But how did these complex molecules organize into structures resembling the first living cells? Next, we examine the spontaneous formation of protocells, or vesicles, from fatty acids, providing a protective environment where these RNA molecules could further evolve and function.
Encapsulation and Protocells
Now that we have complex RNA molecules that can makes copies of themselves, what’s the next step for forming life? Encapsulation, the action of capturing and surrounding something within a container or membrane (which is a wall made out of chemicals) is suggested by astrobiologists as being an important process in forming primitive life. Cells today are bound and regulated by membranes, which are composed of of phospholipids. It is not clear how the first phospholipids formed, but it is possible that the earliest membranes in protocells were first composed of a less complicated lipid connected to hydrocarbon chains.
Protocells can be thought of as simple cell-like structures that form spontaneously from molecules such as fatty acids that were present in the prebiotic environment. For a protocell to form, it requires that it can encapsulate all the molecules needed for that cell to function. However, if you’ve ever heard the old saying “oil and water” don’t mix, you’ll know that certain molecules don’t generally dissolve in water, making them hard to encapsulate within a protocell. So, how do we get different types of molecules to all cooperate together in one protocell?
A type of molecule called an amphiphile may be the solution to our “non-mixing molecules” problem. Amphiphiles are molecules that have a water “loving” head (i.e. part of the molecule that can dissolve in water) and an oil “loving” tail (i.e. part of the molecule can dissolve in oil). This allows it to interact with both oils and waters, helping them co-exist to form protocells. Detergents such as dish soaps are amphiphilic. The hydrophilic head of dish soap will bond with water molecules and the hydrophobic tail will bond with the oils (for example, grease on a pan), therefore allowing the grease to mix with the water and be removed from the pan.
The first protocells developed from even simpler compartments which are composed of a type of molecule called lipids. An early form of protocells, called vesicles, can form naturally and can randomly encapsulate different molecules at different concentrations. Vesicles can be made in a lab!
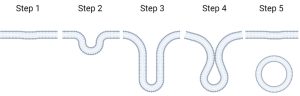
Vesicles can form in a natural environment due to what is known as wet/dry cycling (Figure 2). When an environment is dry, the lipids form sheets (Step 1), however when they get wet again (like after it rains) the sheets can slowly bud off (Steps 2-4) and eventually form vesicles (Step 5) and encapsulate whatever material was between the lipid sheets. This material can include molecules important to the origin of the first life!
Clays like montmorillonite can also catalyze the formation of longer RNA chains by providing a surface upon which lipid molecules can become concentrated, react and polymerize. Chains of up to 50-60 nucleotides have been formed experimentally in this way.
Key Concepts and Summary
The biochemistry for life first emerged on Earth 3-4 billion years ago. Since the fossil evidence of this chemistry has long been erased, some scientists have tried to recreate the process in their labs. One of the most famous experiments was carried out by Miller and Urey in the 1950’s and resulted in spontaneous synthesis of five different amino acids. More studies point to an early RNA world that evolved a molecular code – a precursor of our genetic code – to adapt to environmental conditions.
Review Questions
Summary Questions
- What was the Miller-Urey experiment? How was it set up?
- What did the results of the Miller-Urey experiment imply about the formation of the building blocks of life on the early Earth?
- What does abiogenesis mean? Is there direct evidence for abiogenesis on Earth? Explain your answer.
- What are ribozymes?
- How does the RNA World hypothesis explain how ribozymes could be a pathway to the first life on Earth?
- How has experimental work on synthetic self-replicating systems informed our ideas on the first life on Earth?
- How can protocells naturally form on the early Earth, without the need for proteins?
- How can clay minerals, like montmorillonite, facilitate the process of forming protocells?
Exercises
- Check out the Exploring Origins Project, created in collaboration with Jack Szostak’s lab in 2006.
the emergence of life from nonliving materials
The chemical interactions that happened prior to the emergence of life which eventually became part of living systems.
experiment conducted in 1953 demonstrating that conditions similar to that on the early Earth were conducive to producing amino acids.