18 The Jovian Moons
Although the massive gas and ice giant planets of the outer solar system almost certainly do not harbor any life, some of their large moons are intriguing possibilities. Once presumed to be frigid geologically dead worlds, missions to the outer solar system have shown some of these moons to be dynamic worlds, with active volcanism. Scientists now think that a number of the outer solar system’s moons may harbor liquid oceans. Among these, Europa, Titan and Enceladus have thus far been of greatest interest to astrobiologists.
Learning Objectives
By the end of this chapter, you will be able to:
- Explain how tidal heating provides a source of energy for some of the jovian moons
- Discuss “ocean worlds” and why they may harbor life
- Discuss the environment of Saturn’s moon Titan and what types of life could exist
- Describe how lakes beneath ice sheets on Earth could help scientists learn how to explore icy moons
Tidal Heating
A quick glance at Figure 1 shows that the moons of Jupiter (at least the four largest) look quite different from the Earth’s moon. The moon on the far left is Io and it is the most volcanically active world in our solar system. Io is almost the same size as our Moon: the Moon has a radius of 3476 km, and Io has a radius of 3640 km and a mass that is 1.2 times greater than the Moon’s mass. Additionally, Io is nearly the same distance from Jupiter that the Moon is from the Earth. With just these comparisons in mind, we’d expect Io to have been long geologically dead. So, why is Io so different? Where is the energy coming from to drive the active volcanism on Io?
The difference is the size of Earth compared with the size of Jupiter. Jupiter is a behemoth compared to Earth, with 318 times the mass of the Earth and a radius about 11 times larger than that of Earth. This means that the gravitational pull between Io and Jupiter is much stronger than the pull between the Moon and the Earth. But there’s more to the story with gravity. At any given time, one side of Io is closer to Jupiter. This means that the pull of gravity is stronger on this closer side and the net result is that Io is literally stretched out. On its own, this is not enough to explain Io’s energy source, as we know the Moon is geologically dead and also experienced this kind of stretching. This type of force due to stronger gravity on one side of an object is called a tidal force. The difference is that Io is also experiencing gravitational tugs from Europa, Ganymede and Callisto, as shown by the small arrows in part (A) of Figure 2.
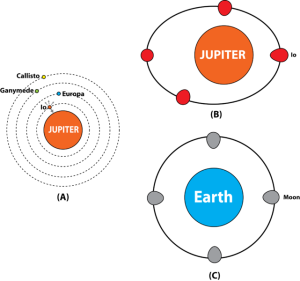
The orbits of Io, Europa and Ganymede are locked into an orbital resonance. For every 1 orbit around Jupiter that Ganymede makes, Europa makes 2 orbits and Io makes 4 orbits. As a side effect, the same sides of these moons always face Jupiter (we say they are tidally locked with Jupiter). When Io receives these periodic tugs from Europa and Ganymede, its orbit becomes more elliptical, meaning it deviates from a perfect circle. This is shown in part B of Figure 2 and the animation below, where Io is stretched and squeezed by different amounts as it orbits Jupiter. This means that Io experiences stronger or weaker tidal forces as it orbits Jupiter, and this continued change in its shape leads to friction and heating. Tidal heating is thus the mechanism that supplies Io with a source of heat.
The Moon is also tidally locked to the Earth — we always see the same side of the Moon from Earth. But the Earth’s orbit is not continually changed like Io’s so the Moon’s shape is not changing as it orbits Earth (See part C of Figure 2).
Jupiter’s Potentially Habitable Moons
Io orbits closest to Jupiter (Figure 1) and so experiences the strongest tidal heating from Jupiter’s massive gravity. This effect is so strong that Io is thought to be the most volcanically active body in our solar system. At far right, Callisto shows a surface scarred by billions of years’ worth of craters—an indication that the moon’s surface is old and that Callisto may be far less active than its sibling moons. Between these hot and cold extremes, Europa, second from left, orbits at a distance where Jupiter’s tidal heating may be “just right” to sustain a liquid water ocean beneath its icy crust.
Io, Ganymede, and Callisto
Because of Io’s extreme levels of volcanism, it is very difficult to imagine any kind of life that could survive in this hostile environment. Ganymede is the largest moon in the solar system — it is larger than the planet Mercury — and also experiences some tidal heating from its elliptical orbit around Jupiter. However, an ocean beneath the icy-rock surface of Ganymede would be under a fairly thick crust (150 km) and would be more challenging to explore. Callisto does not receive tidal heating from Jupiter but it is sufficiently large that it may still retain some internal heat and could host a subsurface ocean.
We will not explore Ganymede and Callisto’s possibilities for life further here. However, ESA’s JUICE (Jupiter Icy Moons Explore) mission was launched in April 2023 and is on its way to do exactly that. The JUICE spacecraft will reach Jupiter in 2031…stay tuned!
Europa
Jupiter’s moon Europa revealed itself to the Voyager and Galileo missions as an active world whose icy surface apparently conceals an ocean with a depth of tens to perhaps a hundred kilometers. As the moon orbits Jupiter, the planet’s massive gravity creates tidal heating on Europa, just to a lesser extent than on Io (since Io is closer to Jupiter).
Evidence for a salty water ocean
The first hint that Europa was different from our own Moon came from spectroscopic observations of its surface in the 1950s and 1960s. Recall that we can use spectroscopy — the splitting apart of light from an object — to learn about the chemical makeup of an object. In the case of Europa, the light is reflected sunlight that bounces off the surface of Europa. Water and ice have strong spectral signatures in infrared light, and infrared spectroscopy of Europa’s surface showed that it was covered in ice. Images of Europa’s surface were taken with the Voyager 1 and 2 spacecraft in 1979 and these images showed a cracked icy surface with few craters. The lack of craters suggested that perhaps liquid water exists beneath the icy crust that can upwell and “pave” over any craters, similar to the way lava wells up from Io’s surface and smooths over regions next to the volcanic eruption. Since tidal heating causes volcanic activity on Io, it is consistent that tidal heating could keep any liquid water below the surface of Europa heated.

More evidence for Europa’s subsurface ocean came from the Galileo mission in the 1990s. Galileo took more close-up images of Europa’s surface, adding to the collection taken by the Voyagers, and made measurements of Europa’s magnetic field. Just like the Earth, Jupiter has strong magnetic fields and consequently a large magnetosphere surrounding the planet. It turns out that Jupiter’s changing magnetic field actually creates a secondary (or “induced”) magnetic field around Europa. Magnetometers aboard the Galileo spacecraft mapped out Europa’s magnetic field and showed that it is consistent with a salty, liquid water layer beneath Europa’s icy surface.
Other evidence supporting the existence of the liquid water ocean come from the detection by the Hubble Space Telescope of water vapor plumes erupting from Europa’s surface (this same idea of cryovolcanism is relevant for Saturn’s moon Enceladus below); measurements of Europa’s moment of inertia, which depends on its internal structure, also support the liquid water hypothesis. The video clip below summarizes the evidence for Europa’s global liquid water ocean.
Source of Energy
Europa has probably had an ocean for most or all of its history, but habitability requires more than just liquid water. Life also requires energy, and because sunlight does not penetrate below the kilometers-thick ice crust of Europa, this would have to be chemical energy. On Earth, life near the bottom of the ocean, far removed from any sunlight above, gets its energy from a process called chemosynthesis. Plants on Earth take in energy from the Sun to create their food — this is photosynthesis. Chemosynthesis works similarly to photosynthesis, except that the energy comes not from the Sun but from chemical reactions. The environment at the bottom of Europa’s ocean may be similar to hydrothermal vent communities on Earth’s ocean floor, where life may have gotten its first start.
One of Europa’s key attributes from an astrobiology perspective is that its ocean is most likely in direct contact with an underlying rocky mantle, and the interaction of water and rocks—especially at high temperatures, as within Earth’s hydrothermal vent systems—yields a reducing chemistry (where molecules tend to give up electrons readily) that is like one half of a chemical battery. To complete the battery and provide energy that could be used by life requires that an oxidizing chemistry (where molecules tend to accept electrons readily) also be available. On Earth, when chemically reducing vent fluids (for example, H2S or methane) meet oxygen-containing seawater, the energy that becomes available often supports thriving communities of microorganisms and animals on the sea floor, far from the light of the Sun.
The Galileo mission found that Europa’s icy surface does contain an abundance of oxidizing chemicals. This means that availability of energy to support life depends very much on whether the chemistry of the surface and the ocean can mix, despite the kilometers of ice in between. That Europa’s ice crust appears geologically “young” (only tens of millions of years old, on average) based on the low number of craters and that it is active makes it tantalizing to think that such mixing might indeed occur. Understanding whether and how much exchange occurs between the surface and ocean of Europa will be a key science objective of future missions to Europa, and a major step forward in understanding whether this moon could be a cradle of life.
Future Exploration
Europa is of such great interest to astrobiologists that a NASA mission, called the Europa Clipper, is set to launch in October of 2024. While the Europa Clipper will not land on the surface of Europa and do any drilling (this is still a challenging technological feat, as it would need to drill beneath about 20 km of ice), it will conduct extensive experiments while in close orbit of Europa.
Saturn’s Potentially Habitable Moons
Enceladus
Another tantalizing ocean world is the small (500 km diameter) moon of Saturn, Enceladus. The story of how Enceladus was found to be a world with an icy surface, with a salty water ocean beneath, follows similar lines as the evidence for Europa. Spectroscopy of Enceladus revealed the presence of ice, and Voyager 1 and 2 took the first up-close pictures of its surface. The Voyager photos showed some heavily cratered regions but a very smooth region near Enceladus’ south pole. The Cassini mission began studying Saturn in 2004 and visited Enceladus multiple times before it ended in 2017.
In 2005, the Cassini mission performed a close flyby of Enceladus, and made the remarkable discovery that plumes of gas and icy material were venting from the moon’s south polar region. The Cassini spacecraft eventually flew directly through the plumes in 2015 and detected the presence of water, salts, organic compounds, silicates and hydrogen gas (H2). All of this chemistry is suggestive of interactions between liquid water and rocky material beneath Enceladus’ icy surface. As with Europa, this could point to hydrothermal vents within Enceladus’ subsurface ocean, which is an environment with all of the ingredients for life to form. The plumes are associated with the blue features called “tiger stripes” in the southern part of Enceladus (see the left side of Figure 6). The tiger stripes are fissures on the surface that are a pathway between the surface and the liquid water beneath. The plumes represent a type of geological activity called cryovolcanism. The prefix cryo means cold and this type of volcanism involves icy materials being spewed out, rather than hot lava.
Strong evidence for a global liquid water ocean beneath the surface, rather than a body of water only below the south polar region, came from Cassini measurements of a tiny wobble (called libration) of Enceladus as it rotates on its axis and revolves around Saturn. The size of the libration can only be explained by a global ocean; if the libration was smaller, this would mean that the subsurface layer was at least partially solid rather than liquid.
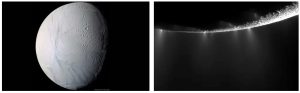
Titan
Saturn’s largest moon Titan was discovered in 1655 by the Dutch astronomer Christiaan Huygens. In the 1940s, Gerard Kuiper used spectroscopy and found that Titan has an atmosphere containing methane. Images of Titan taken by the Voyagers confirmed the atmosphere but were unable to see through the clouds to the surface. The Cassini mission, which was so valuable for learning about Enceladus, also did a (literal) deep dive into Titan: Cassini’s Huygens probe descended through Titan’s atmosphere and landed on its surface in 2005.
Titan’s Atmosphere and Surface
Titan is very different from both Enceladus and Europa. Although it may host a liquid water layer deep within its interior, it is the surface of Titan and its unusual chemistry that makes this moon such an interesting place. Titan’s thick atmosphere—the only one among moons in the solar system—is composed mostly of nitrogen (N2) but also of about 5% methane. Voyager detected hydrocarbons in Titan’s atmosphere, indicating an active chemistry in which sunlight interacts with the atmospheric nitrogen and methane to create a rich mix of organic compounds. In the upper atmosphere, the Sun’s ultraviolet light breaks apart the methane and nitrogen molecules and recombines them into more complex organic compounds that are collectively known as “tholins” (Carl Sagan coined this term). The tholins shroud Titan in an orange haze, as seen in the right panel of Figure 7.

In the atmosphere, the conditions are right for some of the hydrocarbons to condense and fall as rain to Titan’s surface. This methane or ethane rain creates lakes and rivers on Titan’s surface. When combined with an atmosphere and a cycle analogous to Earth’s water cycle, this sounds like a habitable environment! We do need to keep in mind that the lakes are made of liquid methane and ethane and not liquid water; it is far too cold for liquid water to exist on Titan’s surface. On Earth, we follow the liquid water and find life. On Titan, perhaps we will follow the liquid methane or ethane and find life with a different biochemical basis. A radar image of Titan’s lakes, taken by Cassini in 2006, is shown in the right in Figure 7.
The Huygens Probe
In January 2005, the Huygens probe descended to the surface of Titan and relayed data, including imagery of the landing site, for about 90 minutes. You can watch a video about the descent of Huygens to Titan’s surface below (the footage is real and not CGI!):
Huygen’s probe
Video Credit: @NASAJPL
Future Exploration
NASA has selected a new mission to Titan for launch in 2027. Called Dragonfly, this mission is a drone that will fly in Titan’s atmosphere, with emphasis on study of prebiotic chemistry (see details at https://dragonfly.jhuapl.edu/). Other future proposed missions include a balloon operating in the atmosphere and a “boat” floating in one of the Titan lakes.
Life Under the Ice on Earth
What can we expect to find living in an ocean underneath a thick, icy shell? We can gain some insight by seeing what has been found by exploring subglacial lakes — meaning lakes that are buried beneath glacial ice — on Earth. Subglacial lakes exist in Antarctica and many international research stations have been established there, including the Russian Vostok station and the U.S.’s McMurdo Station. Two subglacial lakes in Antarctica, Lake Vostok and Lake Whillans, have been particularly well-studied for the possibility of any life within them. Both are freshwater lakes, with Lake Whillans about 800 m (or 0.5 miles) beneath the ice and Lake Vostok at a deeper 4 km (2.5 miles) below the ice. While these are not an exact analogs to Europa or Enceladus’ oceans, it is still informative to assess the habitability of these types of environments as well as trying out new techniques to drill beneath thick ice. Contamination is also a very big challenge to overcome when drilling through ice.

Lake Vostok was confirmed to exist via radar mapping in the 1990s and is about the size of Lake Ontario. Scientists were able to drill close to the lake’s surface in 2013. Microbes, some previously unknown, were found living inside the ice. While the layer of ice studied is very close to the lake’s surface, this is still not direct evidence of life in Lake Vostok.
Lake Whillans was also drilled into in 2013. Samples of sediments and water were collected and analyzed.
Key Concepts and Summary
The conditions for the chemistry of life might exist on moons as well as planets. The larger moons orbiting Jupiter and Saturn are intriguing because tidal heating could provide an extra source of energy. There is evidence for an ocean of water under the ice mantle surface of Jupiter’s moon, Europa. Since life as we know it requires water, Europa is a key target in our search for life in the solar system and NASA is launching an exploratory mission to Europa. Saturn is twice the distance from the Sun as Jupiter, but plumes of water have been observed coming from the surface of Enceladus (one of the moons orbiting Saturn). Titan is Saturn’s largest moon and the Cassini mission has returned images of a thick atmosphere and pools of liquid methane and ethane on the surface, making Titan a key location for the study of biochemistry in cold environments that makes use of solvents other than water.
Review Questions
Summary Questions
- Explain the process of tidal heating and how it provides energy to some of the jovian moons.
Exercises
- Europa’s Ocean. Describe the three pieces of evidence for a salty liquid water ocean beneath Europa’s icy surface.
- Why is it easier to study what is inside Enceladus’ ocean than what is in Europa (or Ganymede or Callisto’s) ocean?
- Research the moons Ganymede, Callisto and Triton (a moon of Neptune) and discuss the evidence for liquid water and organic molecules on these worlds. Based on what you found, do you think these worlds are habitable? Explain why or why not.