25 Biosignatures and Technosignatures
Traveling to another solar system to visit exoplanets is impossible today and in the foreseeable future. SETI scientists instead search for signs of life by looking for its telltale signature. These signatures can take the form of biological signs of life, like oxygen (O2) for Earth life, or signs of technology, such as the byproducts of chemical pollution from an industrial civilization. A more direct detection of technology could come in the form of an artifact that was deliberately sent our way. In this chapter, we will consider the different forms that these signatures of life can take and consider which type we may expect to find first.
Learning Objectives
By the end of this chapter, you will be able to:
- Explain the concept of a biosignature and describe Earth’s biosignatures
- Discuss how biosignatures can be searched for in exoplanetary atmospheres
- Explain the concept of technosignatures and describe Earth’s main technosignatures
- Discuss some technosignatures that astrobiologists search for from other planets
- Discuss the “cosmic haystack” and how much of it has been searched for signs of other intelligent life
Biosignatures
It is apt to begin a discussion of biosignatures with the adage “Extraordinary claims require extraordinary evidence.” As we look at some possible signatures of life, we cannot immediately conclude that the detection of just one biosignature means we have found other life in the universe. For any potential biosignature detected, absolutely all abiotic sources must be ruled out. False positives can be difficult to identify but an exceedingly critical analysis is in order.
A good place to start in pondering atmospheric biosignatures on other planets is to see what Earth’s atmosphere looks like — and what another civilization would see if they took a spectrum of the Earth. Figure 1 shows the spectrum of Earth’s atmosphere at optical and near-IR wavelengths. The first thing that you may notice is water…and recall “Follow the water!”. This is a sign of liquid water oceans on Earth, so we are indeed excited when H2O is detected in an exoplanetary atmosphere, but water vapor can also be produced naturally (recall that Jupiter and Saturn have water vapor in their atmospheres).
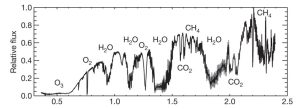
Carbon dioxide is also a byproduct of life on Earth — all animals exhale CO2 and CO2 is released when plants decay. But CO2 can also be produced naturally though volcanic eruptions, and through anthropogenic (man-made) sources such as the burning of fossil fuels or defo restation. To further exemplify this point, Figure 2 shows the atmospheric profiles for Venus, Earth and Mars.
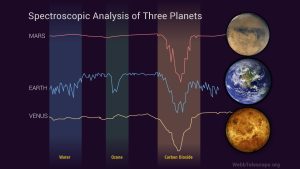
Mars, Earth and Venus all have CO2 in their atmospheres but only Earth shows water and ozone (which is related to O2). Let’s take a deeper look at some potential biosignatures and their abiotic sources. As we’ll see, oxygen alone or methane alone would not be a good biosignature, but the combination of oxygen and methane is a smoking gun for life. At the temperatures and pressures in the atmosphere of Earth, oxygen would react quickly with methane, producing carbon dioxide and water. The simultaneous existence of these elements on Earth occurs only because life is producing these elements and maintaining chemical disequilibrium.
Oxygen
Life on Earth keeps O2 in our atmosphere — it is a byproduct of life on Earth. Ozone — O3 — is formed when an O2 molecule is broken apart and one of the single oxygen atoms combines with another O2 molecule. So, the presence of ozone is a proxy for O2. Of course, just O3 is not enough and a more compelling case would be made if both O3 and another biosignature were detected. For example, ozone and water in our atmosphere point to habitable conditions on the surface.
False negatives need to also be considered in addition to false positives. Just because an atmosphere does not show O2, this does not necessarily mean that there is no life. On Earth, cyanobacteria began putting O2 into our atmosphere perhaps as early as 3.8 billion years ago (stromatolites) but it would not have showed up in a spectrum because it would immediately react with other elements. On a similar note, if there was a very low level of O2, our instruments may not be sensitive enough to detect it. Finding unambiguous signs of life is a tricky game to play.
Methane
Methane (CH4) is another molecule that can be produced both biotic and abiotic sources. Methane is a natural byproduct of volcanic outgassing and, in our own solar system, the lifeless worlds Uranus and Neptune have methane in their lifeless atmospheres. Methane can also be produced geologically in hydrothermal vent communities through a process called serpentinization. Methane is created by life as a waste product. Some animal life, such as cows and sheep, produce methane through microbes in their stomaches. These ancient archaean microbes, called methanogens, take in H2 and CO2 and expel CH4 as waste. Like O2, CH4 will generally not stay in an atmosphere unless it is continuously replenished causing chemical disequilibrium. This is why the topic of methane in Mars’ atmosphere often pops up in the news, as it could be interpreted as a sign of life (but, if the detection is real, is very likely due to permafrost evaporating at the Martian poles).
Methane on its own would not be a strong biosignature but if it was detected with O2 then a very strong case could be made for life, as this combination would be hard to create without photosynthesis.
Sulfur gases
Gaseous molecules containing sulfur are produced by both abiotic and biotic sources on the Earth. Simple sulfur gases such as SO2 and H2S are produced by metabolism but also are produced naturally though volcanic outgassing. A less ambiguous biotic signature is from more complex sulfur cases such as dimethyl sulfide (DMS) and dimethyl disulfide (DMDS). DMS on Earth is created mainly by marine phytoplankton and is also found in microbial mats.
The atmosphere of the exoplanet K2-18 b — a Super-Earth orbiting in the habitable zone around its host star — was recently studied by JSWT. When K2-18 b passed in front of its host star, K2-18, the infrared spectrograph on JWST collected the light from the planet and the star, and was able to separate out the atmosphere of just K2-18b, shown in Figure 3. DMS was detected but further studies will be needed to corroborate this detection.
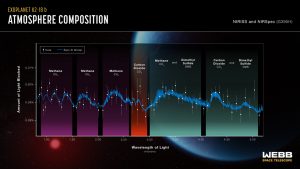
Nitrous Oxide
Nitrous oxide (N2O) is detected in excess (chemical disequilibrium) in the Earth’s atmosphere. It can be created from both abiotic and biotic sources. One biotic source is actually more of a technosignature — the amount of N2O in our atmosphere has risen exponentially since the Industrial Revolution in 1750. It is a byproduct of agriculture and fossil fuel burning. It is also produced by bacteria that use nitrates in the soil and water to create N2O. Nitrous oxide can also be formed naturally by lightning, but the bulk of N2O in Earth’s atmosphere is due to life.
If the detection of N2O is to be considered a biosignature, it must be considered along with other factors about the exoplanet and other gases that are found in its atmosphere.
Vegetation Red Edge
In addition to biosignatures gases in the atmosphere, a planet’s surface can also reveal the presence of life. On Earth, the red edge is a property of chlorophyll in photosynthetic plants, which becomes abruptly reflective at infrared wavelengths as shown in Figure 4. At wavelengths above about 0.7 μm (700 nm, which is right at the edge between red visible light and infrared light), leaves will reflect sunlight and show a sharp increase in the amount of light reflected at this point.
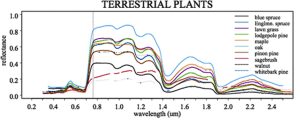
The specific wavelength for the red edge on Earth is a consequence of the properties of the Sun and the intensity of its light at different wavelengths (the most intense light from the Sun being yellow-green). For an exoplanet orbiting an M star, the most intense radiation is in the infrared part of the electromagnetic spectrum, so the red edge could actually be some different color or perhaps a black edge, depending how vegetation responds to the different intensity of light. H.G. Wells considered this and wrote in The War of the Worlds in 1895: “The vegetable kingdom in Mars, instead of having green for a dominant colour, is of a vivid blood-red tint.”
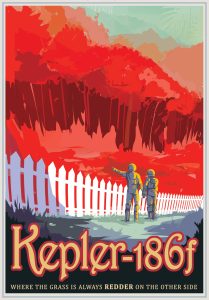
This reflectance property of chlorophyll also keeps plants from overheating, but is also integral to plants growing efficiently through a process called shade avoidance. Plants are capable of detecting a lack of infrared light and this triggers a response in plants that produces longer stems and larger leaves, which allows a plant to capture more sunlight.
Photosynthetic organisms were a critical step in the evolution of aerobic respiration in eukaryotes. Therefore, a similar detection in the atmospheres of other planets would be an intriguing clue for bio-activity. The red edge is a strong signal in the atmospheric spectra from Earth and an easy feature to search for in the atmospheres of other planets.
The image to the left shows an example of an exoplanet, Kepler-186f, that is Earth-sized and falls into the habitable zone around its host star. Kepler-186 is a cool, red dwarf star so any plants that grow there could adapt to their star’s light in such a way that leaves have different colors that differ from those on Earth.
Technosignatures
In 2007, Jill Tarter wrote in her proceedings from the International Astronomical Union’s 2006 General Assembly meeting:
“If we can find technosignatures – evidence of some technology that modifies its environment in ways that are detectable – then we will be permitted to infer the existence, at least at some time, of intelligent technologists.”
This succinct definition encompasses a broad range of possibilities for technosignatures, including some of the biosignatures discussed above that are the result of a technologically advanced civilization’s industrial output.
The first SETI searches were looking for technosignatures. Frank Drake’s Project Ozma in 1960 focused on the 21 cm hydrogen line with the assumption that other technologically advanced civilizations would utilize this wavelength for communication. Dyson spheres and Bracewell probes are both signs of technology. It wasn’t until exoplanets were first discovered in the 1990s that searches for biosignatures in their atmospheres could be realistically considered.
We can imagine many different types of technosignatures, from communication signals using light to entire planetary systems that have been re-engineered to better suit their inhabitants’ needs. It is impossible to know what a Type II or Type III civilization might have the technological capacity to do so we will stay focused on technosignatures that do not require any new laws of physics.
Radio Signals
In the chapter on SETI, we discussed the theory behind radio searches at certain “magic” frequencies such as the hydrogen spin-flip at 1420 MHz. Modern searches cover a broader range of radio wavelengths: Breakthrough Listen searches the range from 1-10 GHz. Of course, it is impossible to predict what frequency another civilization would choose, but we can gain some insight from Game Theory and the concept of Schelling Points. The basic idea of Schelling points, which is explored more deeply in the box below, is that it is worthwhile to consider what strategy your opponent will adopt before beginning a game. For SETI, this translates to thinking about how another civilization would communicate with us, especially if the civilization is also thinking about how we will try to communicate with them.
Want to know more: Schelling Points
Game Theory is the science of strategy. More often than not, the best way to win a game is to have a strategy. But what if you cannot communicate with the other player in a game and the goal is for you both to cooperate to win? There are still ways for the players to come up with a winning strategy by intentionally thinking about what the other person will do and realizing that they will also be thinking about what you will do. The American economist Thomas Schelling wrote about this in his 1960 book called “The Strategy of Conflict.” Schelling focused on political conflict resolution and how to navigate negotiations in a mutually beneficial way. He gave some examples that explicate the idea of what he called focal points.
Imagine you are to meet someone in New York City but you have no plan and you cannot communicate with the other person. You both need to guess where and when to meet. New York City is a big place so random guesses won’t be a successful strategy. [For fun, think about this specific question and come up with an answer. This specific game was carried out in NYC and was successful!] There are some obvious places and times and, so the thinking goes, two rational people will arrive at one of these points.
In his book, Schelling specifically mentions 1420 MHz as a focal point for SETI. Schelling’s book came out just one year after the Cocconi and Morrison paper that suggested 1420 MHz as a frequency that other civilizations like us should be aware of.
We can also consider a Schelling point (Jason Wright has called these Schelling points in the context of SETI, as not to be confused with the other meanings of the term focal point) in time for extraterrestrial communications. We learn a great amount of information by observing exoplanets when they transit their host star. For example, the radius of the planet and how long it takes to orbit its star can be determined with transit data. A civilization at or above our technological level would also realize that they can learn a lot about other planets through transits, so they may deliberately send a communication out in our direction when they know we can see their transit.
Thinking about Schelling points for SETI reminds us that there are also social scientific and psychological aspects to consider.
For more details, see Jason Wright’s Schelling Points page on his blog: https://sites.psu.edu/astrowright/2017/07/09/schelling-points-in-seti/
Activity 1 at the end of this chapter allows you to think about how to approach Schelling Points.
One way to identify an artificially created signal would be an extremely narrow bandwidth. There are limits to how narrow the signal from an astrophysical source can be so artificial signals could be distinguished in this way, although there is still some broadening of the signal that occurs as it travels to Earth (due to the relative motion of the extraterrestrial transmitter and our receiver on Earth).
The main signature of Earth’s technology is through our radio leakage. Earth’s radio and TV transmissions have been traveling away from the Earth for more than 80 years now — meaning any civilizations located up to 80 light years away from us could potentially have intercepted them — and we can add military radar and wifi signals to that list. Could we detect similar radio leakage from another civilization? Breakthrough Listen can get us close with the Meerkat, a precursor to the SKA (Square Kilometer Array).
Another possible way that a technologically advanced civilization might catch our attention is by changing the output of a natural radio source, such as a pulsar. An extremely advanced civilization could change the pulse rate — perhaps have the period flip back and forth between two different times — in a way that could not happen naturally.
Laser Pulse Beacons
Optical SETI searches for communication signals in the form of pulses of laser light. The pulses would need to be communicated in a way that we will notice, perhaps by having them come as bursts of prime numbers (two flashes, three flashes, five flashes, seven flashes, …). This type of communication is a technosignature as the civilization sending the pulses must be advanced enough to have developed lasers.
Megastructures

In 1960, Freeman Dyson described a hypothetical construct that an advanced civilization capable of harnessing all of the energy from its star (a Type II civilization on the Kardashev Scale) would build. These “Dyson spheres,” as they have come to be known, could be made of a network of materials that can absorb the star’s light and transform it into some other type of useful energy. A Dyson sphere could surround an entire solar system. Figure 6 shows an example of a Dyson sphere around Earth in our solar system — the radius of this megastructure would be 1 AU (astronomical unit)! Dyson recognized that a solid shell of material would not be possible to build so instead envisioned a ring of objects in a swarm surrounding the star. Dyson swarms are also frequently discussed, with the objects in the swarm sometimes comprising habitats that contain energy collectors.
How could we detect such a megastructure? The light that is absorbed by the material making up the ring or swarm would be re-radiated as infrared radiation, just as sunlight absorbed by the Earth’s surface is re-emitted as heat. Thus, infrared telescopes could detect this “waste heat” as an otherwise unexplainable excess of infrared radiation. A star with a Dyson sphere around it could also appear to suddenly start flickering or have all of its visible light disappear. The sky can be monitored for this unusual type of star, which would be accompanied by the infrared waste heat.
Transiting Objects
We have already seen the power of using the Transit Method to detect exoplanets. The Transit Method works simply, by measuring the change in brightness of a star to look for dips caused when a planets passes in front of the star, and the results from NASA’s Kepler and TESS missions have been prolific. The same principle and techniques for finding exoplanets can be used to search for technosignatures. (The Transit Method can also be used to search for exomoons, which we discuss elsewhere.)
Megastructures

If a technologically advanced civilization has built a Dyson swarm, this could show up as a series of dips in the brightness of a star. The details of the light curve would depend on how the Dyson swarm is constructed (Figure 7 shows an example of a swarm made of a series of polar rings) but we can easily imagine the brightness dips caused by the material passing in front of its star.
An excellent example of what a transit curve from a Dyson swarm might look like is seen by looking at the real light curve for the star KIC 8462852, also known as Tabby’s Star for the astronomer, Dr. Tabitha Boyajian, who led the team that studied the star’s behavior.
Tabby’s Star
In 2016, a postdoctoral fellow at Yale University, Tabby Boyajian, published a paper on a strange light curve measured by Kepler. The signal was strong but irregular and unlike anything that had been seen before. The light curve for Tabby’s Star was flagged by the citizen science project Planet Hunters, which presented the public with light curves from Kepler data to look for any anomalies. It worked! A paper draft sat on her desk for two years as the Planet Hunter team tried to reason out an explanation – the best idea was that this was a disintegrating asteroid or irregular dust cloud. Failing any certain ideas for the source of the signal, the paper was published in the hopes that someone else would have a better explanation. Almost immediately, Jason Wright at Penn State University suggested an idea: perhaps this was an alien megastructure, like the one suggested by Freeman Dyson. Of course, this idea was picked up in the popular press. Credible news stories with titles such as “I’m STILL Not Sayin’ Aliens. But This Star Is Really Weird” popped up immediately (this particular one is from slate.com and was written by astro-myth-debunker Phil Plait).
The light curve for Tabby’s Star is consistent with a Dyson swarm. The figure below shows some follow-up data taken by an amateur astronomer in 2017-2018 and the large dips in brightness are easy to spot, with the largest dip occurring in March 2018.
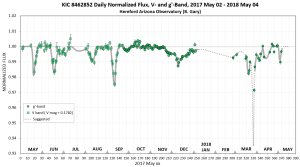
Once again, we note that this is certainly an extraordinary claim and extraordinary evidence is needed. Astronomers quickly applied Occam’s Razor and identified several simpler, non-alien explanations to explain the light curve. An early idea was that there is a system of comets that occasionally occludes the light from Tabby’s star. It is now widely accepted that the dips in Tabby Star’s light can be explained naturally by dust but it is an interesting case study in how sensational claims are disproven. Dr. Boyajian gave a TED Talk about the discovery back in 2016:
Structure Beacons
A Dyson swarm could reveal itself in a light curve found using the transit method. Another type of technosignature that could be detected this way is an object that is not round and has a shape that is designed to create a distinct signature in the brightness data. A couple of examples are shown here, from a 2005 paper that explored this idea:
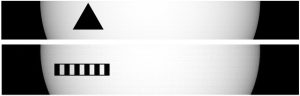
The civilization could place the object deliberately in their transit zone so Earthlings would see it when we are observing their star. We can think of these deliberately placed objects as “structure beacons,” as opposed to laser pulse beacons. These structures could be light collectors, such as those in a Dyson swarm, or perhaps deliberate pulses in an attempt to cloak the civilization’s biosignatures.
Exobelts
The first artificial satellite launched into orbit around the Earth was the Soviet Sputnik I in 1957. Sputnik did not stay in orbit after completing its mission but humans have placed more and more satellites into Earth’s orbit since that time, and over 10,000 are orbiting Earth today. Not all of the satellites are active — some stay up after the mission is complete and these add to Earth’s growing collection of space junk. Objects orbiting Earth can be placed at different altitudes. Some objects, such as Space X’s StarLink internet satellites, are placed in low-Earth orbits. Others, such as weather satellites, are placed in geostationary orbits so that they orbit at the same rate that the Earth rotates and thus are “fixed” at one location over the Earth. These satellites are one of Earth’s technosignature and we can imagine that another advanced civilization could also have “belts” of satellites orbiting its planet.
If a belt of material around an exoplanet was sufficiently wide and far enough from the planet, it could be picked up using the transit method. Such exobelts have been speculated to be in geostationary orbits, placing them high enough to have a detectable signature in the light curve of the star the exobelt is around. Exobelts at geostationary orbit have been called Clarke Exobelts, as a reference to an article written in 1945 by science fiction writer Arthur C. Clarke that put forth the idea of using geostationary orbits for extraterrestrial communication.
Planet-Planet Occultations
Another artifact of our technological civilization that could be detected are the satellites we have sent out to explore our own solar system. Earthlings are in regular radio communication with satellites all over the solar system, from the rovers on Mars to the New Horizons orbiter in the Kuiper Belt, through the Deep Space Network. Perhaps another solar system is carrying out similar planet-to-planet communications with their own network and we could detect these transmissions. Note that the planets do not need to be in the habitable zone — we communicate with satellites orbiting Jupiter and on Mars, neither of which are habitable. The alignment needs to be such that from Earth, there is a straight line to the two planets as they both transit the star (these events are called planet-planet occultations). If you know exactly when these occultations happen, you can either search through existing data or schedule observations to see if any anomalous communication signals are mixed in.
Artifacts (SETA)
The search for extraterrestrial artifacts is sometimes referred to as SETA. The Earth has sent artifacts out to broadcast our existence. While the Voyager Gold Records and Pioneer plaques help to keep the public engaged in SETI, these are not expected to be collected and responded to by another civilization. What about artifacts sent our way from another civilization? These extraterrestrial artifacts of course could take on many different forms and could be intentionally sent for us or we may randomly pick up a piece of their technology.
Concept Check
List a few types of artifacts that an extraterrestrial civilization might send out. For each object, explain how it could be detected by Earthlings. If it cannot be detected, explain why.
Some ideas that have been posited include objects that are placed on the Moon or another place in our solar system, similar to the monolith in the film 2001: A Space Odyssey, that will be activated when we reach a certain level of technology. The Earth’s Lagrange points with the Sun or Moon could also be places that an alien artifact would be placed. Lagrange points are places of gravitational stability, so an object placed there would not drift away (this is why we place some of Earth’s space mission crafts at these points). Perhaps a Bracewell probe could be parked at a Lagrange point and observing Earth.
A piece of alien technology could also drift into our solar system, intentionally or perhaps randomly (as a fanciful example, imagine a piece of the blown-apart Death Star in Star Wars hurtling away from its location in space). Interstellar objects can enter our solar system and two have been detected to date. One of them, 1I Oumuamua (the designation “I” stands for “interstellar”) was previously discussed as an object that was briefly considered to be an alien artifact. However, extraordinary evidence to support this claim has not emerged.
The Cosmic Haystack
SETI is sometimes described as looking for a needle in the cosmic haystack. We can search for these alien needles in different ways but SETI is largely focused on identifying needles that are hidden in a star’s light.
Having made the decision that radio is the most likely means of communication among intelligent civilizations, we still have many questions and a daunting task ahead of us. Shall we send a message, or try to receive one? Obviously, if every civilization decides to receive only, then no one will be sending, and everyone will be disappointed. On the other hand, it may be appropriate for us to begin by listening, since we are likely to be among the most primitive civilizations in the Galaxy who are interested in exchanging messages.
We do not make this statement to insult the human species (which, with certain exceptions, we are rather fond of). Instead, we base it on the fact that humans have had the ability to receive (or send) a radio message across interstellar distances for only a few decades. Compared to the ages of the stars and the Galaxy, this is a mere instant. If there are civilizations out there that are ahead of us in development by even a short time (in the cosmic sense), they are likely to have a technology head start of many, many years.
In other words, we, who have just started, may well be the “youngest” species in the Galaxy with this capability. Just as the youngest members of a community are often told to be quiet and listen to their elders for a while before they say something foolish, so may we want to begin our exercise in extraterrestrial communication by listening.
Even restricting our activities to listening, however, leaves us with an array of challenging questions. For example, if an extraterrestrial civilization’s signal is too weak to be detected by our present-day radio telescopes, we will not detect them. In addition, it would be very expensive for an extraterrestrial civilization to broadcast on a huge number of channels. Most likely, they select one or a few channels for their particular message. Communicating on a narrow band of channels also helps distinguish an artificial message from the radio static that comes from natural cosmic processes. But the radio band contains an astronomically large number of possible channels. How can we know in advance which one they have selected, and how they have coded their message into the signal?
Table 1 summarizes some of these factors that scientists must grapple with when trying to tune in to radio messages from distant civilizations. Because their success depends on either guessing right about so many factors or searching through all the possibilities for each factor, some scientists have compared their quest to looking for a needle in a haystack. Thus, they like to say that factors such as those in Table 1 defines the cosmic haystack problem.
Factors |
---|
From which direction (which star) is the message coming? |
On what channels (or frequencies) is the message being broadcast? |
How strong is the signal (can our radio telescopes detect it)? |
Can we even recognize a message from a completely alien species? Might it take a form we don’t at all expect? |
How much of the cosmic haystack have we searched?
There is a lot of hay (stars) in the cosmic haystack so to estimate how much of it has been searched for alien needles we need to identify some parameters that we can use to quantify this immense space in a robust way. It is reasonable to confine the search to our home galaxy, the Milky Way, for two reasons. First, the finite speed of light prohibits any meaningful intergalactic communication. Also, if we accept the Copernican principle, our galaxy is representative of any other galaxy in the universe, so life in the Milky Way would mean life in other galaxies. We can further confine the haystack to just focus on searches in the radio part of the electromagnetic spectrum. This is where most previous searches have looked and Breakthrough Listen also focuses on the region between 1-10 GHz. In 2010, Jill Tarter estimated what fraction of the haystack has been searched and she reported her result with this memorable analogy:
“So our search of the 9-dimensional haystack is equivalent to sampling about 1.6 cups of water from the Earth’s oceans. If you were looking for fish instead of extraterrestrial intelligence, I don’t think that you would conclude that there are no fish in the ocean after this meager sampling!”
Jason Wright at Penn State published an updated estimate in 2018 and he found that the 1.6 cups could be increased to a large hot tub or small swimming pool. On the one hand, this is progress! On the other, we still have a lot of space to search.
Key Concepts and Summary
The goal of SETI searches is the detection of technological signals from other civilizations. Recognizing that simple organisms were altering the atmosphere of Earth for billions of years before our radio telescopes appeared, astronomers can cast a wider net in the search for life by looking for the impact that life might have on the spectra of atmospheres of other planets – so called biosignatures. For example, the combination of strong oxygen markers together with methane is a signature of chemical disequilibrium on Earth that requires ongoing biological respiration. There could also be technosignatures that are being transmitted by pulsed lasers or transiting megastructures that harvest more light from their host stars. We have only sampled a tiny bit of the universe – the equivalent of sampling 1.6 cups of water from all of Earth’s oceans.
Review Questions
Summary Questions
- What is a biosignature? Give some examples of Earth’s biosignatures.
- How do SETI scientists search for biosignatures in planetary atmospheres?
- What is a technosignature? Give some examples of Earth’s technosignatures.
- What types of technosignatures do scientists search from exoplanets? Give at least three examples and explain each one briefly.
- What is the “Cosmic Haystack”?
- How much of the cosmic haystack has been searched so far? Explain your answer.
Exercises
- Think of our Milky Way Galaxy as a flat disk of diameter 100,000 light-years. Suppose we are one of 1000 civilizations, randomly distributed through the disk, interested in communicating via radio waves. How far away would the nearest such civilization be from us (on average)?
Activities
- Group activity. Read the box on Schelling points. Imagine that as a class, you are asked to come up with a time and place to meet on your college’s campus. You cannot communicate with each other at all. Individually, come up with a time and place for the meeting. Now compare all of the suggested times and places. Do they all match?
- The Cosmic Haystack. Table 1 lists some factors that must be considered before carrying out a SETI search in the radio part of the electromagnetic spectrum. Can you think of any additional questions that need to be considered, in order to maximize the search strategy?
when a result is reported as true but is actually false
when a result is reported to be false (negative) but is actually true (positive)
a relatively small range of wavelengths covered for a signal