Chapter 11: Emotion and Affective Neuroscience
11.3: Basic Emotions and Brain Circuitry
Facial Expressions
Although best known for his theory on evolution, naturalist Charles Darwin published about many other topics in biology, including emotion. Darwin suggested that emotional responding is similar across different cultures, and to some extent, even in nonhumans. In his view, the main purpose of emotional expressions are to communicate survival cues: a relaxed expression conveys safety, while a fearful expression promotes alertness, since danger may be nearby. Darwin also suggested that we also gain survival information from non-human behaviors, for example, a hissing snake or a snarling lion is an immediate threat that should cause fear or other avoidance behaviors.
More recently, the American psychologist Paul Ekman expanded on Darwin’s theory, and suggested that there are seven basic universal emotions: Anger, contempt, disgust, fear, happiness, sadness, and surprise. Like Darwin, Ekman theorized that all humans, regardless of culture, use similar facial expressions. To test this hypothesis, Ekman visited a remote village in Papua New Guinea, where he studied a population that was isolated from any other cultures. As predicted, these people made the same facial responses in reaction to various emotional circumstances as they do in other parts of the world. In 1972, Ekman published his theory of universal facial expressions.
Ekman’s team also created a series of photographs of actors portraying six major emotion (Anger, contempt, disgust, fear, happiness, sadness, and surprise). This Ekman 60 faces (EK-60F) test has been widely used to assess whether people recognize facial emotions. We have learned that people with major depressive disorder or borderline personality disorder are less able to detect happiness in others, and people with dementia or Parkinson’s disease identify emotions as being less intense than typical participants.
Ekman also developed the Facial Action Coding System (FACS), which uses facial anatomy to differentiate the characteristics of different expressions. For example, features of a happy face include the flexing of the zygomaticus major and orbicularis oculi muscles, which produce an upward turn of the corners of the mouth and a rising of the cheeks. Other facial features, such as head movement, eye movement, and larger physical movements are also scored, and are also used to help identify emotions. The FACS can be used to formally describe why some smiles appear as genuine (a Duchenne smile, where the smile reaches the muscles of the upper part of the face) while others look fake or forced (a non-Duchenne smile, characterized by the turn of the corners of the mouth without much change to the top of the face).

Cognitive neuroscientists who study emotion often struggle to find appropriate stimuli to elicit emotions in an ethical way in their human participants. However, emotional faces are frequently used in affective neuroscience studies because they have been shown to elicit somewhat similar emotions in the observer, i.e., seeing pictures of happy faces tends to make you feel more happy. Many emotional faces activate the amygdala, and disgusted faces activate the insula cortex, the part of the brain that processes information about taste.
Fear: The neural system of freezing and fleeing
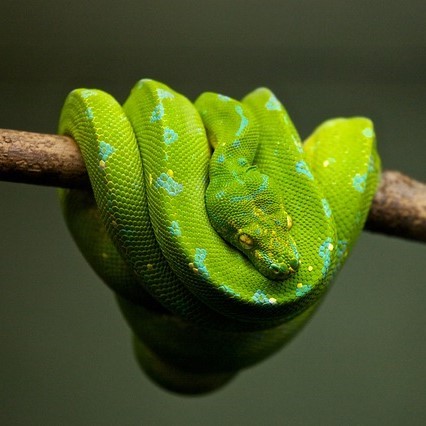
Nearly everyone has experienced the prototypical fear response: Imagine reading a book when you see a spider skittering along the wall. Suddenly, you feel your heart racing, your breathing increase, your mouth is dry and and your palms sweaty. You probably won’t notice the dilation of your pupils or the change in liver activity and digestion, but these also happen when you experience this flight or flight response caused by sympathetic nervous system activation. But on closer inspection, you might realize there was no spider at all – just an small piece of brown fuzz picked up by a draft. Within minutes, your body’s physiology returns back to normal.
This anecdote points out a few important features about the fear response. First, the onset of the fear response is quick, and so is the dissipation of the fear. Second, it is triggered by exposure to a perceived threat, regardless of whether the stimulus is a genuine threat or not (the overwhelming majority of spiders are clinically harmless to humans!). Third, the fear response is greatly modified by knowledge and experience – an spider expert would recognize that the spider is a harmless house spider and would, instead of fear, display curiosity, interest, boredom, or other emotions. On the other hand, someone who has been bitten by a dangerous spider and sent to the hospital when younger would have a much stronger physiological response.
Fear is likely the most evolutionary ancient emotion, and is highly protective. When encountering a hungry mountain lion, faces displaying the traits of fear (enlarged eyes, flared nostrils, and a slightly open mouth accompanying a gasp) would signal to others nearby that a threat is nearby, which helps initiate heightened alertness and the appropriate fight-or-flight response.
Amygdala and Fear
The amygdala is strongly implicated in both positive and negative emotional memory formation. However, the role of the amygdala in fear responses has been particularly extensively studied. Roughly the shape and size of an almond, the amygdala is part of the temporal lobe. Generally, the amygdala is subdivided into several nuclei, including the basolateral amygdala, central nucleus, and cortical nucleus. A useful nonhuman model for studying emotional learning is the fear conditioning paradigm. In this test, a rodent is put into a cage. Occasionally, a sound and light would activate. Shortly after, an unpleasant foot-shock would be delivered to the animal. Over time, the healthy animal learns that the tone and light precede the shock to the foot, and upon future presentation of these stimuli, they will freeze. If the amygdala is lesioned, however, they freeze less, meaning they do not acquire the emotional memory associated with the stimuli. We also know that monkeys become fearless when their amygdalae are removed. Amygdala lesions have been used as a last resort treatment for patients with temporal lobe epilepsy or with psychiatric symptoms of pathological and dangerous aggressiveness. These psychosurgery strategies have been variably successful, but they often have high complication rates and upwards of a 4% mortality rate. These treatments are rarely used today (Lim, 2021). Deep brain stimulation may offer a less intrusive and therefore less risky therapeutic approach (Lim, 2021).
Patient SM is a notable case study of a person who does not experience the fear response. Born with an extremely rare genetic condition called Urbach-Wiethe disease, Patient SM progressively developed calcification in her amygdala bilaterally, causing cell death and amygdala degeneration. Like other people with Urbach-Wiethe disease, she had no severe significant cognitive deficits, except for the inability to experience fear. In one test, she was shown a variety of emotionally charged videos, then asked to rate the intensity of each clip with respect to different emotions. SM found clips from America’s Funniest Home Videos to be just as funny as the control patients, and she found the clips of disgusting toilets to be just as repulsive. But, when presented with clips depicting ghost hauntings or suspenseful serial killers on the loose, SM rated these stimuli as being non-fearful. Other studies challenged Patient SM with more concrete threats. Researchers brought SM to a pet store, where she asked to handle the snakes. She was stopped by an employee before she put her hand into a tarantula cage out of curiosity. The researchers also took her to the Waverly Hills Sanatorium haunted house, where she bravely led a group of strangers through the house populated by actors dressed as monsters and ghosts. Although she did not display any fearful behaviors, such as hesitation to walk through the darkened corridors, patient SM reported the sensation of exhilaration and enthusiasm, akin to riding a roller coaster.
She also was asked to recall some of her past real-life, fear-provoking experiences, such as when she was attacked in a domestic violence incident or was held up by a stranger at knife point in a public park. In none of these cases did she ever report feeling fear, although she was upset and angered at the situation. The destruction of her amygdala seemed to make her resilient against PTSD: the day after being threatened with a knife to her throat, she walked past the very same park bench.
Slight stimulation of the fear-related areas in the brain causes animals to freeze, whereas intense stimulation causes them to flee. The fear circuit extends from the central amygdala to the periaqueductal gray in the midbrain. These structures are sensitive to glutamate, corticotrophin-releasing factor, adreno-cortico-trophic hormone, and several different neuropeptides. Benzodiazepines and other tranquilizers inhibit activation in these areas (Panksepp & Biven, 2012).
Perhaps because fear is so important to survival, two pathways send signals to the amygdala from the sensory organs. When an individual sees a snake, for example, the sensory information travels from the eye to the thalamus and then to the visual cortex. The visual cortex sends the information on to the amygdala, provoking a fear response. However, the thalamus also quickly sends the information straight to the amygdala so that the organism can react before consciously perceiving the snake (LeDoux et al., 1990). The pathway from the thalamus to the amygdala is fast but less accurate than the slower pathway from the visual cortex. Damage to the amygdala or areas of the ventral hippocampus interferes with fear conditioning in both humans and nonhuman animals (LeDoux, 1996). More recent research shows that the amygdala of the nonhuman primate can be divided into 13 nuclei and cortical areas (Freese & Amaral, 2009). These regions of the amygdala perform different functions. The central nucleus sends outputs involving brain stem areas that result in innate emotional expressions and associated physiological responses. The basal nucleus is connected with striatal areas that are involved with actions such as running toward safety.
Rage: The circuits of anger and attack
Fear and anger are two very closely related emotions. Anger or rage is an arousing, unpleasant emotion that motivates organisms to approach and attack (Harmon-Jones et al., 2013). The anger spectrum runs from low (irritation) to high (rage), and from quick (lashing out) to persistent (vengeful). Strong anger can provoke anti-social behavior such as violence (Lim, 2021). Anger can be evoked through goal frustration, physical pain, or physical restraint. In territorial animals, anger is provoked by a stranger entering the organism’s home territory (Blanchard & Blanchard, 2003). The neural networks for anger and fear are near one another but separate (Panksepp & Biven, 2012). They extend from the medial amygdala, through specific parts of the hypothalamus, and into the periaqueductal gray of the midbrain. The anger circuits are linked to the appetitive circuits, such that lack of an anticipated reward can provoke rage. In addition, when humans are angered, they show increased left frontal cortical activation, supporting the idea that anger is an approach-related emotion (Harmon-Jones et al., 2013). The neurotransmitters involved in rage are not yet well understood, but the neurotransmitter and neuromodulator Substance P (also involved in pain and stress) may play an important role (Panksepp & Biven, 2012). Other neurochemicals that may be involved in anger include testosterone (Peterson & Harmon-Jones, 2012) and arginine-vasopressin (Heinrichs et al., 2009). Several chemicals inhibit the rage system, including opioids and high doses of antipsychotics, such as chlorpromazine (Panksepp & Biven, 2012).
Environmental factors such as childhood maltreatment or expectations are partially responsible for how a person responds to a particular anger-provoking stimulus. Internal homeostatic conditions also influence the anger response, just as how low blood sugar increases aggression and drives negative or hateful feelings in the face of a challenge (the portmanteau “hangry” was added to the Oxford Dictionary in 2018). Neurobiological factors also contribute to the anger response. In addition to amygdala circuits, regions in the frontal cortex decrease activity during acts of aggression, suggesting that frontal circuits actively inhibit the limbic system, which drive our more “primitive” responses. Altered frontal cortical action may therefore account for one reason why two different people would react to the same anger-provoking stimulus in different ways (Lim, 2021).
Disgust
The insula contributes to interoception, detecting the internal state of the body and conveying that information for processing. In functional imaging studies, the insula is involved in the recall or many different emotional stimuli, especially those emotions that have a sensory component. Notably, the insula is strongly implicated in the emotion disgust. For example, a patient is placed in an fMRI scanner while breathing through a mask, which allows the experimenters to change the smells that are perceived. Patients are then given pleasant smells (such as passion fruit, pear, or mint), a neutral smell, or unpleasant smells (like ethyl-mercaptan or isovaleric acid, which smells like skunk or body odor, respectively). There is increased activity of the anterior insula in response to the unpleasant smells but not the pleasant smells. The insula also responds to social cues related to disgust as well. When a patient in an fMRI sees a video of a person smelling something unpleasant and reacting with a “disgusted” face (the closing of the nostrils and curling of the upper lip), their anterior insula likewise increases in activity just as if they had smelled it themselves (Lim, 2021).
In addition to sensory stimuli, feelings of social repugnance (unwarranted violence, murder) or moral disgust (incest) also increase insula activity.
Atypical insula activity is implicated in behavioral disorders. For example, insensitivity to disgust can lead to squalor-dwelling conditions (sometimes seen in excessive hoarding or late cognitive decline), which puts those people at heightened health risks due to regular exposure to unsanitary conditions. Substance use disorders, PTSD, and suicide attempts have all been associated with atypical insula activity (Lim, 2021).
Desire: The neural systems of reward-seeking
One of the most important affective neuronal systems relates to feelings of desire or the appetite for rewards. Researchers refer to these appetitive processes using terms such as “wanting” (Berridge & Kringelbach, 2008), “seeking” (Panksepp & Biven, 2012), or “behavioral activation sensitivity” (Gray, 1987). When the appetitive system is aroused, the organism shows enthusiasm, interest, and curiosity. These neural circuits motivate the animal to move through its environment in search of rewards such as appetizing foods, attractive sex partners, and other pleasurable stimuli. When the appetitive system is underaroused, the organism appears depressed and helpless.
Much evidence for the structures involved in this system comes from animal research using direct brain stimulation. When an electrode is implanted in the lateral hypothalamus or in cortical or mesencephalic regions to which the hypothalamus is connected, animals will press a lever to deliver electrical stimulation, suggesting that they find the stimulation pleasurable. Other regions in the desire system also include the amygdala, nucleus accumbens, and frontal cortex (Panksepp & Biven, 2012). The neurotransmitter dopamine, produced in the mesolimbic and mesocortical dopamine circuits, activates these regions. It creates a sense of excitement, meaningfulness, and anticipation. These structures are also sensitive to drugs such as cocaine and amphetamines, chemicals that have similar effects to dopamine (Panksepp & Biven, 2012).
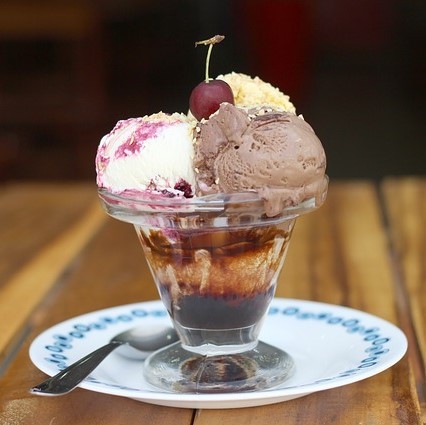
Research in both humans and nonhuman animals shows that the left frontal cortex (compared to the right frontal cortex) is more active during appetitive emotions such as desire and interest. Early researchers noted that persons who suffered damage to the left frontal cortex developed depression, whereas those with damage to the right frontal cortex developed mania (Goldstein, 1939). The relationship between left frontal activation and approach-related emotions has been confirmed in healthy individuals using EEG and fMRI (Berkman & Lieberman, 2010). For example, increased left frontal activation occurs in 2- to 3-day-old infants when sucrose is placed on their tongues (Fox & Davidson, 1986), and in hungry adults as they view pictures of desirable desserts (Gable & Harmon-Jones, 2008). In addition, greater left frontal activity in appetitive situations has been found to relate to dopamine (Wacker et al.,, 2013).
“Liking”: The neural circuits of pleasure and enjoyment
Surprisingly, the amount of desire an individual feels toward a reward need not correspond to how much he or she likes that reward. This is because the neural structures involved in the enjoyment of rewards are different from the structures involved in the desire for the rewards. “Liking” (e.g., enjoyment of a sweet liquid) can be measured in babies and nonhuman animals by measuring licking speed, tongue protrusions, and happy facial expressions, whereas “wanting” (desire) is shown by the willingness to work hard to obtain a reward (Berridge & Kringelbach, 2008). Liking has been distinguished from wanting in research on topics such as drug abuse. For example, people who are addicted often desire drugs even when they know that the ones available will not provide pleasure (Stewart et al., 1984).
Research on liking has focused on a small area within the nucleus accumbens and on the posterior half of the ventral pallidum. These brain regions are sensitive to opioids and endocannabinoids. Stimulation of other regions of the reward system increases wanting but does not increase liking and, in some cases, even decreases liking. The research on the distinction between desire and enjoyment contributes to the understanding of human addiction, particularly why individuals often continue to frantically pursue rewards such as cocaine, opiates, gambling, or sex, even when they no longer experience pleasure from obtaining these rewards due to habituation.
The experience of pleasure also involves the orbitofrontal cortex. Neurons in this region fire when monkeys taste or merely see pictures of desirable foods. In humans, this region is activated by pleasant stimuli, including money, pleasant smells, and attractive faces (Gottfried et al., 2002; O’Doherty et al., 2001; 2002; 2003).
Love: The neural systems of care and attachment
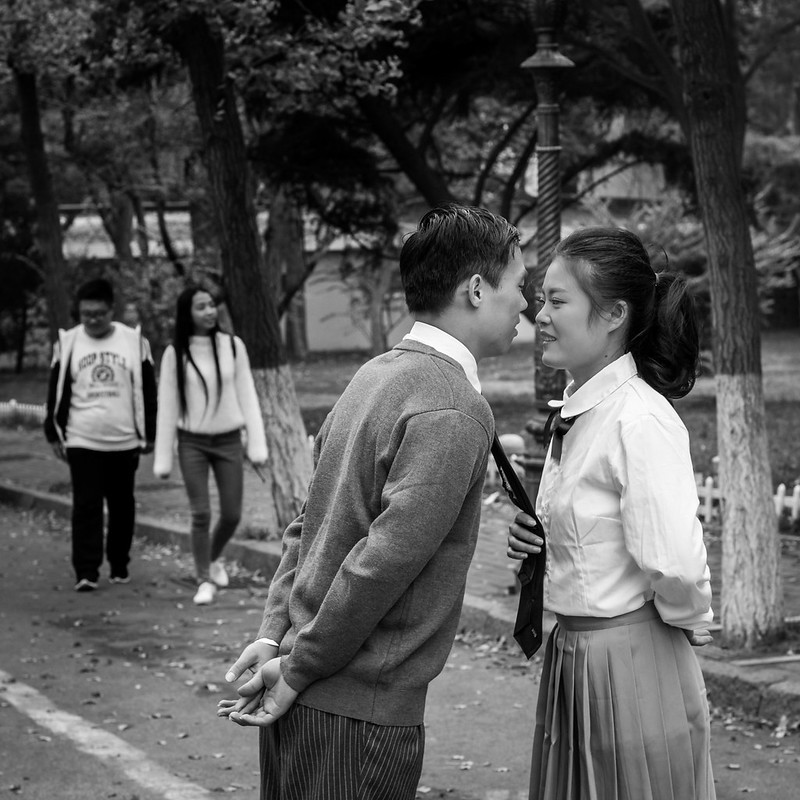
For social animals such as humans, attachment to other members of the same species produces the positive emotions of attachment: love, warm feelings, and affection. There are several unique forms of love, each resulting in different behavioral outcomes. For example, romantic love drives physical attraction, lust, and sexual activity. Parental love, on the other hand, encourages self-sacrifice and hyper-attentiveness towards a newborn. The amygdala plays a role in these behaviors because it sends important messages to the hypothalamus, the brain areas that controls the endocrine system. Oxytocin (OT) plays a significant role in the development and maintenance of prosocial behaviors, acts such as trust, compassion, and empathy, all actions that enhance interpersonal relationships. For example, increased blood levels of OT is seen in new couples compared to unattached singles, and OT release happens during orgasm, which may contribute to romantic attachment. OT signaling increases dramatically during childbirth and triggers milk letdown in lactation, which strengthens the mother/child relationship. Interestingly, while OT generally strengthens the social bonds between people, it promotes antisocial behaviors against those not perceived to be within one’s own social group. Disorders of the OT system are believed to contribute to autism spectrum disorder and psychopathy, two complex conditions characterized partly by social impairment. Some studies have examined the therapeutic use of nasal OT for a variety of psychiatric conditions, but the studies have been unable to demonstrate strong clinical effects despite success in nonhuman animal models (Lim, 2021).
Dr. Helen Fisher, an anthropologist and a leader in the field of romantic love research, suggests that this kind of love can be divided into three closely interconnected components, lust, attraction, and attachment. These three are guided in part by different signaling pathways, and lead to somewhat different behavioral outcomes (Lim, 2021).
Exercises
Check out this video of a tour of the brain areas involved in romantic love
Lust
Lust (or libido) refers to a very strong desire for sexual gratification. These behaviors are largely driven by the actions of the sex hormones testosterone, estradiol, and progesterone, released downstream of activation of the HPG axis. As hormones, they are synthesized from cholesterol and circulate through the bloodstream to influence the body in many ways. Both testosterone and estradiol contribute to sex-seeking behaviors in men and women, where increasing testosterone levels drive up sexual desire.
In the brain, the sex hormones strongly influence the medial preoptic area (mPOA) of the anterior hypothalamus. The mPOA contains a sexually dimorphic area, the part of the brain that exhibits the biggest morphological difference between males and females: in humans, it is about twice as large in males with double the number of neurons throughout childhood and early adulthood. In the rat, it is up to 8 times larger in males than females, and if this area is lesioned, rats exhibit decreased motivation to engage in sex. The amygdala also plays a significant role in mediating lust, and lesions may either result in hypersexuality (Kluver-Bucy syndrome) or a decrease in responding to socially-derived sex cues.
Attraction
Attraction is characterized by high energy investment and preoccupation towards a small number of people. From an evolutionary perspective, attraction may have developed to discriminate between multiple reproductive partners, allowing the focusing of limited resources towards fewer partners.
In Fisher’s theory, attraction is strongly related to the action of dopamine and norepinephrine. In humans, the reward circuitry discussed above is involved in feelings of love. Fisher presented pictures of a patient’s romantic partner to them and identified increases in the blood flow using fMRI to dopaminergic midbrain areas such as the ventral tegmental area and the striatum. This finding compares with imaging studies that observed increases in blood flow to insula, premotor, and hypothalamus as well as striatum in response to highly erotic, sexual imagery. These studies suggest that romantic love and lust have different driving neural structures underlying these behaviors. Norepinephrine increases to boost attention, alertness, and energy, which accounts for the exhilarated feeling you may feel when spending time with a potential partner (Lim, 201).
Attachment
Attachment is the long-term accompanied by feelings of comfort and emotional stability. Attachment also contributes to behaviors that maximize offspring survivability, such as sharing parenthood responsibilities and protectiveness towards offspring. The major neurochemical drivers of this form of love are oxytocin and vasopressin (Lim, 2021).
Parental love
Parental love refers to instinctive affection towards one’s offspring. Parental love behaviors include nurturing (collecting and sharing resources), protecting (promoting aggression against “intruders”), and preparing one’s young for their adult life. In evolutionary theory, parental love serves to improve the odds of passing of one’s genes through the following generation.
Many behaviors related to mammalian motherhood are accompanied by changes in neural activity. Nursing, for instance, is feeding behavior that is regulated through a positive feedback cycle. Offspring suckling activates the mother’s somatosensory afferents. Through a series of oxytocin-dependent circuits across the hypothalamus, suckling ultimately increases lactation through the milk letdown reflex. Oxytocin increases accumulation of milk in the mammary glands (bottom right), which encourages increased suckling. sensory inputs such as the sounds of a crying baby can also trigger this reflex. Sometimes, just thinking about the baby can induce letdown.

The brain changes to facilitate nurturing. For example, after childbirth, the auditory areas of rodents rewire to become more sensitive to high frequency sounds. This adaptation allows the mothers to better detect the ultrasonic vocalizations that are emitted by offspring when they are distressed or hungry. Olfactory areas also change in order to become more sensitive to the particular odorants given off by their young, allowing them to better identify their offspring. In humans, these olfactory changes result in decreased aversion towards traditionally aversive stimuli (urine or fecal matter) when they originate from their children.
Important regions for maternal nurturing include the dorsal preoptic area (Numan & Insel, 2003) and the bed nucleus of the stria terminalis (Panksepp, 1998). These regions overlap with the areas involved in sexual desire and are sensitive to some of the same neurotransmitters, including oxytocin, vasopressin, and endogenous opioids (endorphins and enkephalins) (Hove & Martinez, 2024).
The gray matter in the midbrain near the cerebral aqueduct.
Almond-shaped neural structure that is primarily responsible for regulating emotional responses, especially fear.
A part of the diencephalon that works as a gateway for incoming and outgoing information.
The part of the brain that processes visual information that is located in the back of the brain.
Part of the diencephalon. Regulates biological drives with pituitary gland.
The frontal cortex contains four main gyri.
A region of the basal forebrain located in front of the preoptic region.
A region of the frontal lobes of the brain above the eye sockets.
A part of the anterior hypothalamus.
A band of fibers that runs along the top surface of the thalamus.
Originating from within an organism. For example, endogenous opioids such as endorphins are produced in the brain.