Chapter 9: Learning and Memory
9.4: Cellular Mechanisms of Learning
In the late 1800s, around the time when Golgi and Ramon y Cajal were engaged in intense debate about the organization of the nervous system, many neuroscientists came to a strange observation: the weight of the brain increases dramatically over the first 10 years of life, but not much more after that. Even though we learn lots of new facts and make lots of new memories in adulthood, the brain itself doesn’t grow in size. So how is it possible to store new knowledge if the brain is not making many new neurons?
Most likely, new pieces of information are held in the connections between cells, not just in the cells themselves. If our estimate of 150 trillion synapses per adult brain is correct, then it is possible that we could store all the knowledge and memories that we collect over our lifetime through some combination of activity across certain connections.
The activity at the cellular level is believed to take place on at least three different levels enabling us to build, store, and retrieve memories.
- Encoding refers to the ability for brain circuits to store some piece of information. In real life, you are presented with countless stimuli simultaneously. Imagine walking down a busy street, and think of the number of different sights, smells, and tactile stimuli you experience. Storing memories is an energetically costly process, and we are limited in the fact that all of our sensory inputs cannot possibly get encoded. Instead, evolution has preferred to encode stimuli that are most salient pieces of information, such as perceptual cues associated with predators. Alternatively, information that we pay strong attention to can get encoded more strongly, like when we repeat a phone number to ourselves until we have a chance to write it down. It is also easier to encode novel information that “builds” on previous bits of knowledge, or information that is closely related to other well-established information, which is why analogies are such an effective way to learn new facts.
- The process that enables memory storing is called consolidation, which makes the memory more permanent. In 1949, psychologist, Donald O. Hebb, offered an explanation for how changes in synapses could possibly lead to a phenomenon as complex as learning. His theory can be summed up in the phrase:“Cells that fire together, wire together.”In Hebb’s framework, repeated activity at a synapse within a circuit of neurons acts as a reinforcer signal that strengthens this synapse for future communication, making the next incoming signal more robust. A cellular process called reverberation is thought to be the mechanism that allows for consolidation. Reverberation is the process by which networks of neurons fire repeatedly. Each time that circuit is activated, the strength of the network is increased, meaning that it becomes easier for that circuit to be activated in the future. Hebb also implies the inverse is true: when cells do not fire together, they weaken their connection (“use it or lose it”). A lifetime of memories can be stored across a wide distribution of neurons, by fine tuning synaptic connections, strengthening some and weakening others.
I
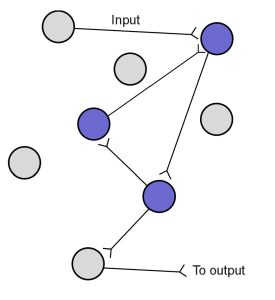
Throughout the process of consolidation, memory traces are thought to become more represented in the neocortex and less dependent on the subcortical structures like the hippocampusor amygdala. Recall Patient HM’s temporally graded retrograde amnesia, where he had lost declarative memories in the two years leading up to this surgery, and yet his memories from the long past were maintained. This finding suggests that some aspects of declarative memory consolidation depends at least partially on medial temporal lobe and HPC for a period of time, maybe up to two years, before those memories get stored in the cortex more permanently. However, we cannot conclude how long consolidation takes in healthy humans based on findings from HM, who had pervasive and frequent seizures, which might have negatively impacted memory consolidation before the surgery.
Consolidation seems to occur predominantly during sleep. Studies suggest that declarative memory is particularly enhanced during non-REM sleep, while procedural memory is enhanced during REM sleep. Studies investigating sleep consolidation are often done by letting participants learn or perform behavioral tasks, after which they are deprived of a specific phase of sleep. To wake participants at the right moment, researchers commonly use EEG signatures, which are unique for different phases of sleep: sleep deprivation early in the night denies non-REM slow wave sleep, while late-sleep deprivation decreases time spent in REM sleep. Some speculate that the visual images we sometimes experience during dreaming are a consequence of consolidation processes, but it is inconclusive as to what role dreaming plays in memory formation.
Long-term memory can be tested in a variety of ways. You could be asked to memorize a list of 50 words and then to write down or say as many as you can remember, this kind of test is called free-recall. However, if you were prompted with some of the categories of the words, e.g., animals or spices. You would likely perform much better at retrieving those memories in this cued-recall test. Multiple choice tests are a form of cued-recall test.
Retrieval is not a passive function. When a memory is retrieved, it is reconsolidated, which is an act similar to replaying the activity of the circuit. During this reconsolidation, it is possible that some aspects of the memory are emphasized, while others are lost. This is one reason why we often experience false memories. A dysregulation of this reconsolidation process may lead to the symptoms seen in post-traumatic stress disorder, where the negative emotional components of a traumatic memory are exaggerated rather than being blunted.
Special populations of neurons
An individual memory is likely distributed widely across several different parts of the brain. However, there are a few special populations of neurons mainly in the MTL that contribute to highly specific types of memories.
Place cells
Place cells are a special population of pyramidal cells of the hippocampus. These neurons increase their firing activity when the animal is in a particular location in an environment, indicating that they contribute strongly to location and navigational memory. There is no apparent topographical arrangement of these place cells, meaning that adjacent areas of an environment do not necessarily activate adjacent hippocampal place cells. The place cells, when firing at the right times, help the animal create a spatial map of their surroundings.
Grid cells
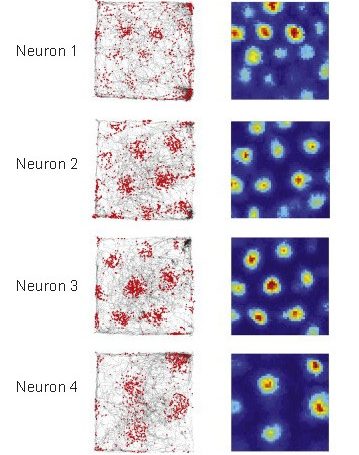
Grid cells are located in the entorrhinal cortex, the main input structure to the HPC. Closely related to the place cells described above, grid cells increase their firing properties periodically when an animal is at an intersection of a “grid” in a wide-open, previously-explored environment. The grid itself is roughly hexagonal, and spans the whole environment an animal is in. The overlap of multiple grids gives the animal an idea of the surroundings. The scientific description of grid cells earned three scientists a Nobel Prize in Physiology or Medicine in 2014.
Jennifer Aniston neurons
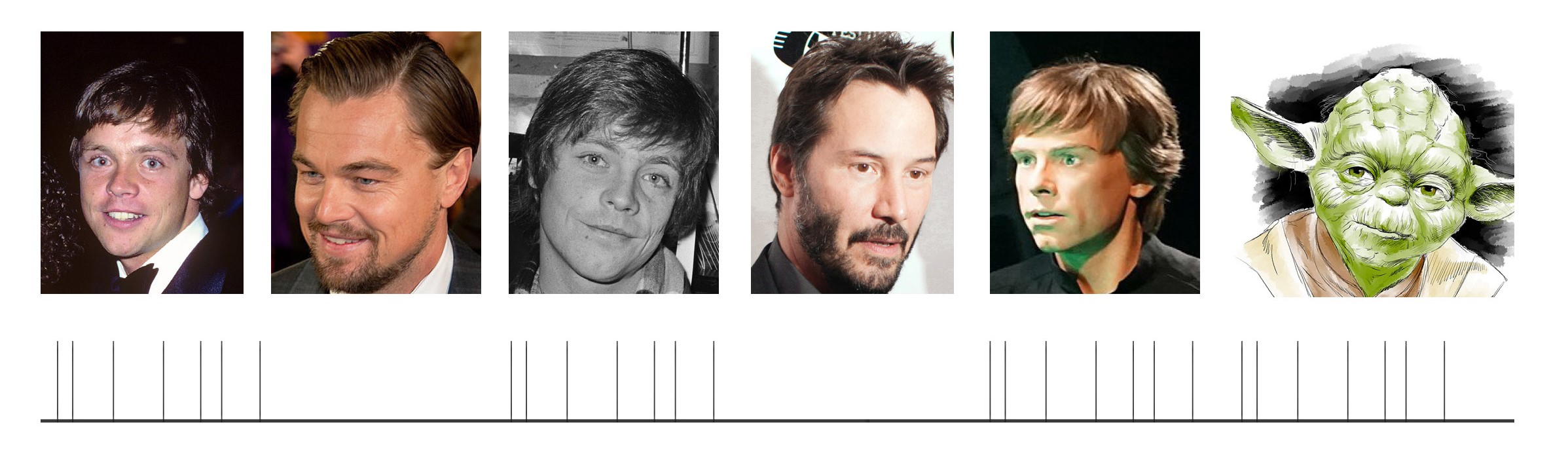
https://commons.wikimedia.org/wiki/File:Retrato_del_Maestro_Yoda.jpg https://commons.wikimedia.org/wiki/ File:Leonardo_DiCaprio_2014.jpg https://commons.wikimedia.org/wiki/File:Keanu_Reeves_2014.jpg
The “Jennifer Aniston” neurons, also called concept cells, are a series of cortical neurons in the temporal lobe that increase their firing exclusively in response to highly-specific stimuli, such as the idea of Jennifer Aniston, Halle Berry, or the Tower of Pisa (based on a person’s experience). These concept cells respond to much more than just pictures: for example, a Luke Skywalker neuron that responded to a picture of young Mark Hamill (the actor who played Luke Skywalker) will also respond to text that reads “LUKE SKYWALKER” and the sound of a person saying “Luke Skywalker”. Although this particular neuron probably won’t fire in response to pictures of athlete Manu Ginobili or actress Marilyn Monroe, the neuron might fire in response to pictures of Yoda or Darth Vader, indicating that the neuron may encode an even broader concept, such as “Star Wars characters” or “Jedi”, or is a part of a network that encodes concepts related to the Star Wars franchise.