Chapter 5: Development of the Brain and Nervous System
5.5: Stages of Neuronal Development
As discussed in previous chapters, the brain is made up of neurons and glial cells. Neurons, also called nerve cells, are electrically excitable cells that transmit signals called action potentials to other neurons and are considered the fundamental units of the brain and nervous system (Ludwig et al., 2022). Neurons communicate information about sensations and movements, and process information within the brain. Glial cells, or neuroglia or simply glia, are the other type of cells found in the nervous system. Glial cells are considered supporting cells, and help neurons complete their function for communication. We discuss six main types of glial cells. Four of them are found in the CNS and two are found in the PNS. Figure 5.11 provides a visualization of the different types of glial cells and Table 5.1 defines their common characteristics and functions.

Glial Cell Types By Location and Function
CNS Glia | PNS Glia | Function |
Astrocyte | Satelite cell | Support |
Oligodendrocyte | Schwann cell | Insulation, myelination |
Microglia | – | Maintenance of neural networks |
Ependymal cell | – | Creating cerebrospinal fluid |
Neuronal Proliferation
Neuronal development includes several stages. Once the neural tube has closed, the first stage of neuron growth, known as neural proliferation, begins to occur in the ventricular zone of the neural tube. Roughly four weeks into embryonic development, neurogenesis begins, such that cells of the neural tube begin to increase significantly in number (i.e., proliferate). The cells produced during neural proliferation will eventually develop into neurons that integrate into the neural circuitry that give rise to the central nervous system (brain and spinal cord). The vast majority of neurons in the human telencephalon (i.e., cortex, hippocampus, basal ganglia, etc.) are generated before birth. Extensive neurogenesis does occur after birth in other regions of the brain (e.g., cerebellum). But all in all, the bulk of neurogenesis (i.e., the 86 billion neurons) in the CNS occurs between 4th week post-conception to 18 months after birth—this means that about 4.6 million neurons are generated every hour during this early developmental period (Silbereis et al., 2016).
During neural proliferation, the cells being formed are neural stem cells. There are two basic types of stem cells, pluripotent and totipotent cells. Pluripotent cells can give rise to all of the cell types that make up the body, whereas multipotent cells are more limited than pluripotent cells. Given that pluripotent stem cells can give rise to all cell types that make up the body, scientists have begun focusing on how pluripotent cells can be used in regenerative cell-based treatment strategies to counter a wide-range of diseases including diabetes, spinal cord injury, and heart disease. In regard to CNS development, neural stem cells are a special type of pluripotent cell, in that they only generate radial glial cells, which eventually give rise to the neurons and glial cells of the central nervous system. In summary, neural proliferation is the process by which billions of cells are generated, and certain cell types (i.e., neural stem cells), have the capability of differentiating into the neurons and glial cells that will develop into the central nervous system.
Neuronal Migration
Newly formed neurons may remain where they are and continue to divide, or may migrate to other parts of the nervous system. Neuronal migration refers to the process by which neurons travel from their original location to a new target location. For neurons of the central nervous system, neural migration remains within the confines of the neural tube, whereas for neurons of the peripheral nervous system, neural migration may take place across different neural regions (Purves et al., 2001). During the migration period, neurons remain immature, and still lack fundamental neuronal characteristics such as axons and dendrites. Ultimately, neuronal migration is supported by sophisticated molecular and cellular signaling that results in pulling and pushing the immature neuron to its appropriate target location.
In general, migration tends to follow an inside-out pattern, where neurons travel from the inside of the neural tube, also known as the ventricular zone, outwards toward their target location. This migration can be classified into two modes: 1) radial migration, and 2) tangential migration. Radial migration, long seen as the primary mode of neuronal movement in the cortex, occurs when neurons are guided by radial glial cells to migrate toward the surface of the brain following the radial pattern of the neural tube and ultimately establish the layered organization of the neocortex (Marin et al., 2003; Wong, 2002). The second mode of neuronal movement, tangential migration, occurs when neurons move to the surface of the central nervous system (or orthogonal to the direction of radial migration). Of note, these two migration methods are not mutually exclusive, as some neurons may alternate from radial to tangential movement along the course of their migration to their target location (Marin et al., 2010).
Additionally, the mechanisms of migration are interesting. One method, called somal translocation, involves an extension that reaches out from the soma of the immature neuron to lead it on its journey to its target location. Both radial and tangential migration can occur through somal translocation. A second method is called glial-mediated migration. This involves the immature neuron “hopping onto” an extended glial cell. This immature neuron “pulls” itself up the glial cell to its target location. Radial migration occurs according to glial-mediated migration.
By the end of migration, neurons are aligned in such a manner that enables them to acquire specific functions, interact with other neurons, and eventually give rise to neural circuits that make up the human nervous system; a process known as aggregation. Aggregation is thought to be supported by cell-adhesion molecules, which are located on the surfaces of cells. Cell-adhesion molecules are able to recognize identical or different cell types and subsequently adhere to molecules on other cells (Jaffe et al., 1990; Takeichi, 1988). Gap junctions are also thought to support migration and aggregation processes. Gap junctions are clusters of communication channels between neighboring cells that link the cytoplasm of two cells and facilitate the exchange of ions and metabolites such as glucose, which subsequently promotes biochemical coupling between the two cells (Mese et al., 2007). Ultimately, through supportive mechanisms such as cell-adhesion molecules and gap junctions, neurons are able to interact and coalesce to give rise to the neural circuitry that makes up the human nervous system.
Neuronal Differentiation
After reaching their target location, the immature neurons begin differentiation — acquiring distinct neuronal characteristics, such as dendrites and axons, which allow neurons to communicate with other neurons via synapses.
Synaptogenesis
Ultimately, synaptogenesis — the ability for neurons to communicate with one another leads to the establishment of functional neural circuits that make up the adult nervous system. In fact, if neurons do not synapse with other cells during development they will die as they are simply not needed. Neuronal cell death, which refers to the elimination of neurons in the nervous system, occurs extensively during development and is actually helpful for supporting brain development. This type of neuron death is called Apoptosis it refers to active, programmed cell death to maintain appropriate development (Khalid & Azimpouran 2023). One example of apoptosis can be seen after neuronal proliferation, when excess neurons and immature neurons must be selectively eliminated in order to enable adequate neuronal connectivity and support the maturation of functional networks (Hollville et al., 2019).
Early research showed a pattern in synapse formation during brain development such that there is first a rapid overproduction of synapses, followed by a programmed elimination of non-functional synapses, which eventually brings the overall number of synapses down to adult levels (Huttenlocher, 1979; Huttenlocher & Dabholkar, 1997; Shonkoff & Phillips, 2000). This synapse-formation pattern emerges at different timescales for different brain networks—synapse formation related to sensory processes peaks first, followed by language-related processes, and finally higher-order cognitive functions (Figure 5.12). Relevant to neural efficiency, one hypothesis proposes that excess synapses during adolescence in frontal lobe regions, such as the prefrontal cortex, may render information processing less efficient in those brain regions (Blakemore, 2012). In other words, during adolescence the brain may require many synapses to carry out cognitive processes. However, as these neuronal connections and functional networks become refined throughout development, the brain may require fewer synapses to carry out the same cognitive processes. In this way, the brain is hypothesized to shift toward prioritizing the most efficient synapses, which may ultimately lead to more efficient cognitive processing.Synapses are constantly being rewired throughout our lives as we learn new things — the function of the brain to change with experience is called neuroplasticity.
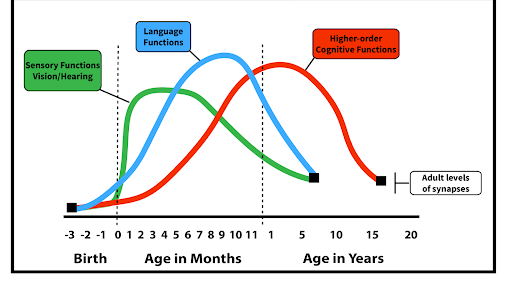
Adult Neurogenesis
Until about 25 years ago, the prevailing view was that new neurons could not be generated in the human brain after birth. However, a landmark research study showed that regions of the adult brain, such as the hippocampus, are able to generate new neurons throughout adulthood (Eriksson et al., 1997). The generation of new neurons in the adult brain is referred to as adult neurogenesis. However, adult neurogenesis does not appear to take place in all parts of the brain. Neurogenesis has been most consistently observed in two regions: 1) the subventricular zone of the lateral ventricles and; 2) the subgranular zone in the dentate gyrus of the hippocampus (Ribeiro & Xapelli 2021). Additionally, of the neurons that are generated during adulthood, many neurons do not survive and as a result, cannot integrate into existing neural circuits. While multiple studies have demonstrated evidence of adult neurogenesis, the topic remains fairly controversial in the field. Some studies suggest that 700 new neurons are generated in the adult hippocampus every day, while other studies suggest that adult hippocampal neurogenesis is undetectable or may not exist at all (Sorrel et al., 2018; Spalding et al., 2013). Nonetheless, given the potential clinical implications of new neurons throughout adulthood, and their potential for possibly preserving cognitive function, future research will continue exploring the mechanisms that support neurogenesis especially in adulthood.
The fundamental units in the brain that transfer information from the brain to the rest of the body.
Non-neuronal cells that support and insulate neurons. Important for supporting typical brain function and protecting neurons.
The mass production of cells. Most prominent during early embryonic development.
The process by which new neurons are formed in the brain.
Self-renewing cells that ultimately generate neurons or glial cells, which give rise to the entire nervous system.
A long threadlike part of a neuron that carries electrical impulses away from the cell body and to other neurons
A branch-like structure of the neuron that extends from the cell body.
The most common mode of neuronal migration in which neurons migrate in a radial pattern to ultimately establish the different layers of the cortex.
Another mode of neuronal migration in which neurons migrate tangentially and may emerge as a result of interactions with axons, astrocytes, or independent of interactions with other cells. Does not rely on glial cells for migration.
The stage of neuronal development after migration where different classes of neurons coalesce to form different nervous system structures.
A subset of cell surface molecules that are involved in the binding of cells with other cells.
A type of cell junction in which neighboring cells are connected through intercellular channels. Also facilitates the transfer of molecules directly from the cytoplasm of one cell to another cell.
Specialized junction through which neural signals are transmitted between neurons.
The process of programmed cell death to eliminate unwanted cells.
The process in which new neurons are generated in the adult brain.