Chapter 8: Circadian Rhythms and Sleep
8.4: The circadian rhythm
Almost every living organism that we know of on Earth exhibits some sort of cyclic pattern of activity that closely matches the rising and setting of the sun. The discovery of 24 hour patterns of behaviors began in 1729 with the French scientist Jean-Jacque d’Ortous de Mairan, who documented the movement of the Mimosa pudica plant. This unique organism was chosen since the plant exhibits heliotropism, light-seeking movements. In particular, this plant opened up the leaves in the daytime to capture sunlight, then closed at night, minimizing predation. When the plants were put into a dark room with no exposure to sunlight, to his surprise they still opened and closed their leaves in time with the clock. Mairan concluded that the plant did not change its behavior in response to light, but rather in response to some internal 24-hour clock. His work laid the foundations for future chronobiologists, scientists who study day- night dependent periodic phenomena in living beings.

Any behavior or physiological measure that intrinsically cycles on a 24-hour pattern is said to be a circadian rhythm. The word circadian comes from the Latin words circa- meaning “around”, and diem meaning “day”. Compare this with an ultradian rhythm, any cycle that is faster than 24 hours, such as the cycling between deep sleep and REM sleep every 90 minutes. Alternatively, compare with infradian rhythms, patterns that are longer than 24 hours, like the 4-week-long human menstrual cycle.Although we mostly think of the circadian rhythm in the context of sleep and wake, many other physiological measures fluctuate reliably throughout the day. Blood pressure peaks at 11 AM, making the morning the time with the highest risk for cardiac events. Body temperature dips late in the evening, putting the body into a low-energy state that helps promote sleep. Withdrawal reflexes peak around midnight, hunger-driving hormone production rises before lunch and dinner, attention is usually highest in the morning – all manner of behaviors that rise and fall depending on the time of day can be said to be a part of a circadian rhythm.
Bizarrely, even organisms with a life span shorter than a full day still exhibit 24 hour cycling patterns of circadian rhythm-like behaviors. Cyanobacteria, or blue-green algae, are capable of a process called nitrogen fixation, where they convert atmospheric nitrogen into organic compounds like amino acids. These organisms are capable of asexual reproduction every 6 hours or so, but they still have nitrogen fixation patterns that align with daily patterns of light and dark.
Circadian rhythms on a behavioral level
Luckily, a person’s circadian rhythm is not permanent. Anyone who has traveled overseas to a different time zone for more than a few days has experienced that uncomfortable sensation called jet lag, where a person experiences psychological symptoms such as difficulty concentrating and mood swings, and physical symptoms like daytime fatigue, insomnia, and gastrointestinal distress (nausea, constipation, or diarrhea). Jet lag happens when there’s a mismatch between the internal environment and the signals that the brain receives from the outside world. If you flew east from Chicago to Cairo, for example, your circadian clock will be off by 7 hours. When your internal “Chicago” clock is telling you to start getting sleepy around 11 PM, the sun will be rising in Cairo, as the locals are starting to wake up. You may be eating when you’re not hungry or laying down in bed when you’re not sleepy, and this mismatch contributes to jet lag.
However, with a few days of adjustment, you will be able to overcome jet lag. You will start sleeping as the sun sets, you will get hungry at the same time as the Egyptians, and your physiological measures will start to align with your time zone. This adjustment is only possible because our circadian rhythms are entrainable, meaning they are able to change and fit the surroundings.
Figure 8.11 Traveling to a part of the world where day and night misalign with your internal circadian rhythm can cause jet lag. Image by Arek Socha from Pixabay
Our circadian rhythms entrain in response to zeitgebers, the German word for “time givers”: environmental cues, such as increased light exposure when the sun comes up, or social cues, such as increased sensory input from heightened activity of the people around you. A rise in the neurohormone melatonin is an important signal that contributes to helping the brain entrain, which is why taking a melatonin supplement late at night when in a new time zone may help someone get over jet lag more quickly. If sunlight is a trigger that helps a person entrain their circadian rhythm to new environments, what would happen if a person is completely isolated from sunlight? In other words, what does a free-running circadian rhythm look like? One of the early documented case studies addressing this curiosity was conducted by a French cave explorer named Michel Siffre. In 1972, Siffre (voluntarily) spent six months deep in a Texan cave to evaluate what would happen to a person completely isolated from zeitgebers. At the end of the experiment, he found his circadian cycle was much longer than 24 hours, and very unpredictable – some of his so-called days would consist of being awake for 36 hours and asleep for 12. A more rigorous scientific study, conducted in a group of people living in an underground bunker with unchanging lighting conditions for several days estimates the typical free-running circadian cycle to be close to 26 hours. In other words, a person absent from external cues begin to fall asleep and awaken 2 hours later each day.
Free-running circadian disruption is a potential issue for scientists aboard the International Space Station, who experience about 16 sunsets and sunrises per day, since the orbiting space vessel completes one trip around the earth every 90 minutes. In order to minimize the negative effects of jet lag on the researchers aboard, NASA has the inside of the vessel set to an artificial 24-hour cycle. Bright blue LEDs illuminate the cockpit in the “daytime,” while dim, red-shifted wavelengths are used in the evening to induce sleepiness.
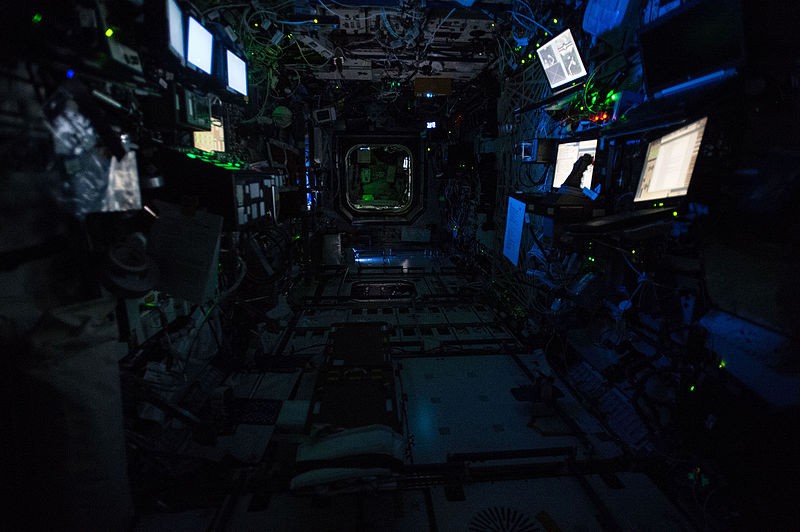
Circadian rhythms on a molecular level
The circadian cycle functions on the molecular scale at the level of cellular transcription. In the mid 1980s, the gene period was discovered in the genome of the fruit fly, Drosophila melanogaster. Normal fruit flies are no exception to 24-hour cycles of behavior, as they generally exhibit periods of wakefulness that are paralleled by the rising and setting of the sun. But, if this period gene was mutated, there was an unusual change in the sleeping habits of the flies. Some of them slept on a 29-hour cycle, some had a shorter rhythm at 19 hours, and some had no predictable sleep-wake pattern whatsoever.
Later, it was discovered that another gene was related to the cycle of sleep and wake, called timeless. This gene codes for a protein called TIM, which interacts closely with the protein coded by period, the protein PER. When PER and TIM interact with each other, they form a dimer (a pair of molecules) with the ability to enter into the nucleus, bind to a specific sequence on the genome, and prevent further transcription of both PER and TIM. Therefore, the paired proteins function as a negative feedback regulatory system.

The TIM protein, however, is degraded by light, so during the daytime, the concentration of TIM in the cell is very low. As a result, the PER protein is left by itself, where it can no longer repress transcription. The cells, without the active repression of transcription, then proceed to create more protein. Once night falls, light no longer breaks down TIM, and TIM begins to accumulate again, forming the dimer with PER, which prevents further protein transcription. The cycle repeats itself the next morning. Since behavior can be driven or modified based on protein levels, the capacity for an organism to change transcriptional activity on a 24-hour cycle suggests that genetic level changes can possibly influence the activity of the whole organism. These gene transcription-level changes were discovered in Drosophila in 1990, and three scientists, Hall, Rosbash, and Young, were recently awarded the 2017 Nobel Prize in Physiology or Medicine.