Chapter 7: Depth perception
7.2. Binocular Disparity
If you close one eye and align your finger with an object on the other side of the room or outside the window and then switch which eye is open, you will notice that what you were looking at seems to jump relative to the position of your finger. This demonstrates the principle of binocular disparity. Each eye has a slightly different view of the world but our brain is able to put hem together into a single image. It is the property of binocular disparity that allows us to see the world in 3-D, which is also known as stereoscopic vision. Most of us have probably seen a 3-D movie or used a VR headset or a children’s Viewmaster toy at some point in our lives. These also work by using the principle of binocular disparity. Pictures or movies are shot using two separate cameras spaced at about the same distance apart as our eye. Then the two pictures or movies are superimposed on each other. Most stereo display systems require some way to ensure that the images can be presented to a person’s left and right eye separately in order to recreate a normal level of visual disparity. For 3-D movies like IMAX-3D, we have to wear glasses to see the 3-D effects. If polarized filters were used to shoot the movie, then we need to wear polarized lenses with filters that isolate the left and right eyes. Another way of doing this is to use two different colored films and using matching filters in the glasses to isolate the left and right eye images (see Figure 7.8). About 5% of people cannot see 3D effects at all because they do not have stereo vision. If someone is unable to see the 3D effect at all, they may be stereo-blind. The most common reason for this is that one eye has a visual problem that was not corrected early in childhood and resulted in amblyopia.
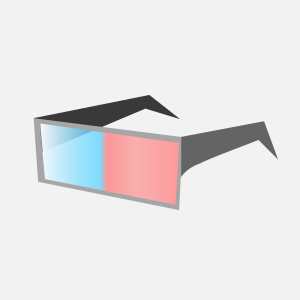
Learn more about stereoblindness by watching the video linked here and included below.
https://www.youtube.com/watch?v=x237dl4pnYc
When considering how we can see in 3-D, it is helpful to define some basic terms. Imagine if we could fit one retina exactly on top of the other. Each point where they touch is considered a pair of corresponding points. One example of a pair of corresponding points in the retina are the foveas. They are in the same location in each eye. We can also draw an imaginary circle through the point we are looking, which is called the horopter (see Figure 7.9). If we look directly at an object – say a tree at point D, it will stimulate each fovea at point D in each eye. In our peripheral vision we will notice that objects at A, B, C, E, F and G seem the same distance away from us as the tree we are looking at. This is because they are on the horopter too, which means that they also stimulate other corresponding points. So, objects on the horopter stimulate corresponding points and create zero disparity. In contrast, imagine there is a pigeon on the grass at point P. The pigeon is in front of the horopter and so if we keep looking at the tree, the image of the pigeon stimulates non-corresponding points on the retina – E in the left eye and C in the right eye. Now there is binocular disparity. We were to close our right eye and only look at the tree with the right eye, we would notice the pigeon would be on the left of the tree and vice versa with the left eye. This is called crossed disparity. Our brain uses this information to tell us that the pigeon is in front of where we are looking. If the pigeon flew behind the tree and we did the same thing over, we would now perceive the pigeon in uncrossed disparity – the right eye would see the pigeon on the right of the tree we are looking at and the left eye would see it on the left of the tree. The greater the angle of disparity, the further away the pigeon would be from the tree we are looking at. Though it is possible that if the angle becomes too large our brain is unable to fuse the two images, and we would see two pigeons!
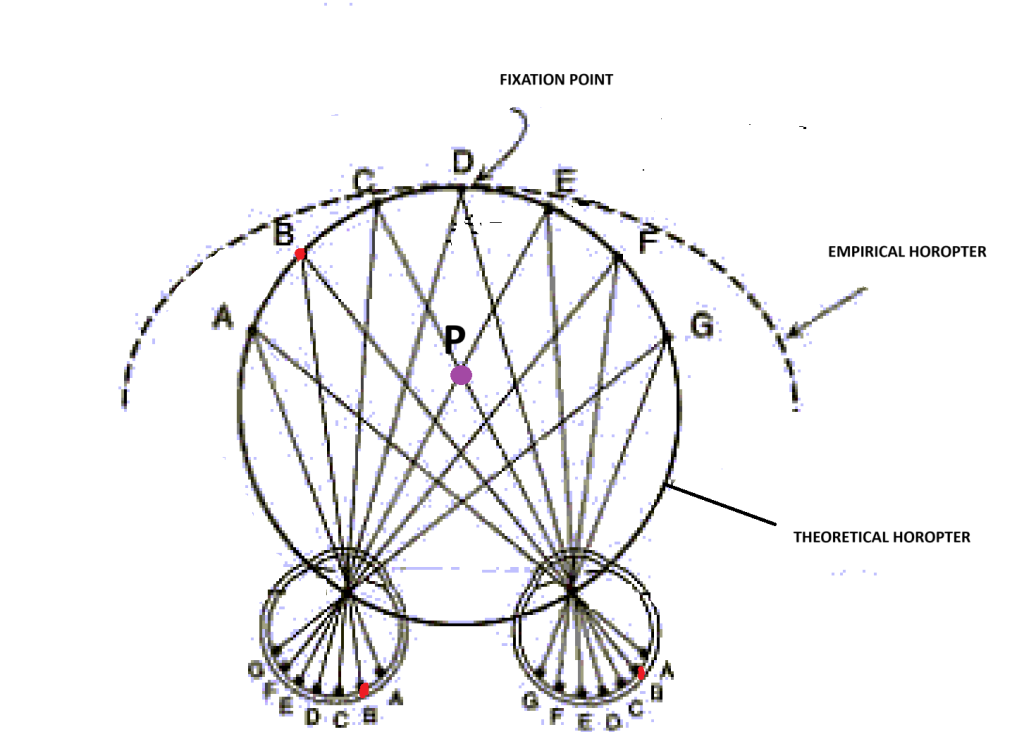
In Figure 7.10, we are essentially doing the same thing, we are looking at the tree but we are aware of our finger in front of us. We can see that if we look only with our left eye, the finger is on the right of the tree, but is on the left when we look with our right eye. Remember this crossed disparity message is what the brain uses to let us know our finger is in front of the tree. In the primary visual cortex (V1), we have a number of disparity neurons, each has a preference for the type of disparity (crossed or uncrossed) and the amount (angle of disparity).
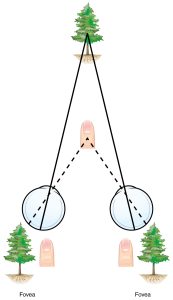