58 7.1 How Memory Functions
Western cognitive psychologists have historically viewed memory as a computer-like information processing system (Figure 7.2). We get information “into” our memories via a process called encoding—energy from the outside world is converted by our sense organs into neural energy and is sent to the brain. Thus, our experiences of the world are represented or coded in terms of patterns of neural activity. The information needs to be stored in such a way that we can retrieve it when needed. However, memory does not work like a video recorder; information is modified during each stage of processing—encoding, storage, and retrieval. Therefore, our memories often do not provide a wholly accurate representation of our external world. We will return to this theme when we discuss memory errors later in the chapter.
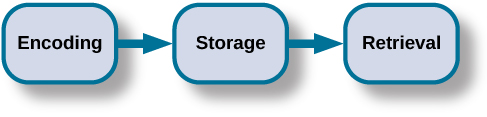
Information-processing models of memory suggest that we have three different types of memory stores, each of which retains information for a different length of time. When we are studying for a test, our goal is to store information permanently, which requires that it reaches long-term memory (LTM). According to Atkinson and Shiffrin’s popular model of memory (Figure 7.3), if we want information to be retained permanently, it has to pass through three distinct stages: sensory memory, short-term memory, and finally long-term memory (Atkinson & Shiffrin, 1968). Information gets re-encoded at each stage of the model.
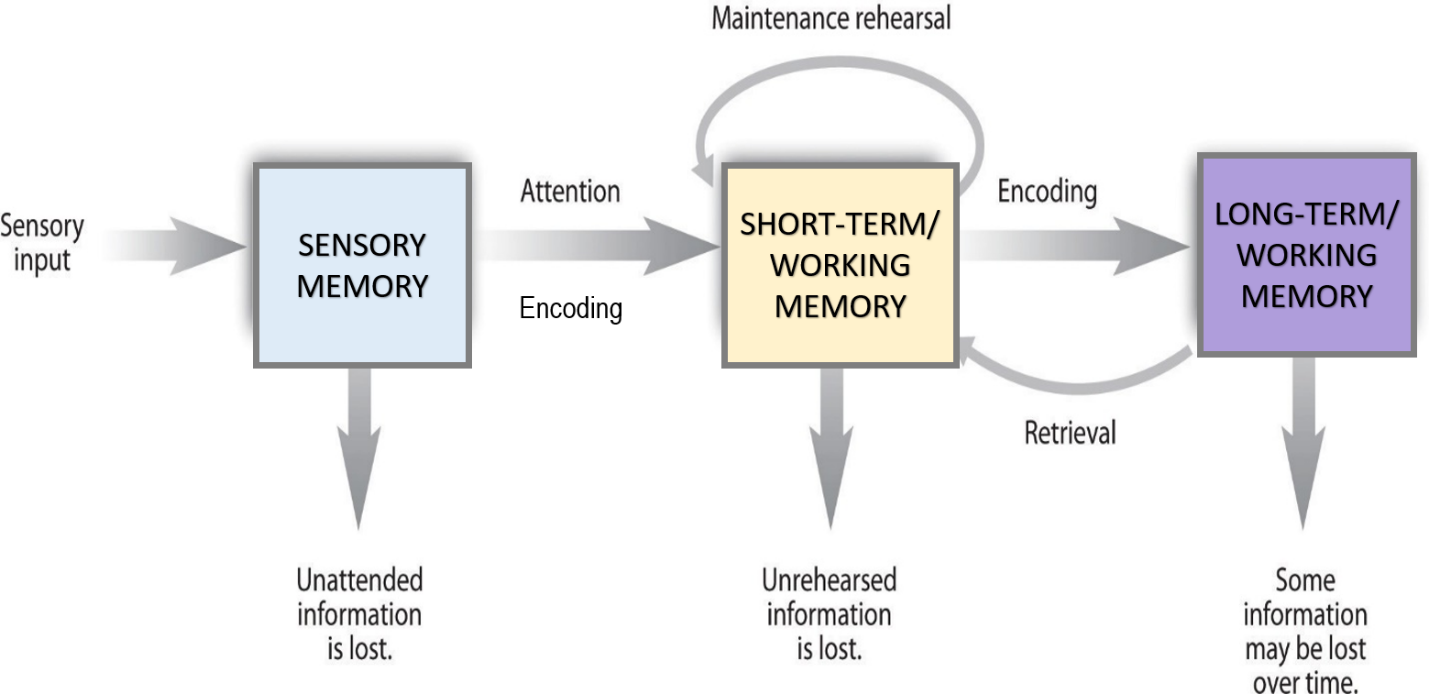
Sensory Memory
In the Atkinson-Shiffrin model, information from the environment is processed first by our various sensory systems (vision, hearing, taste, etc.) and this information is held very briefly in sensory memory. Visual sensory memories (commonly referred to as iconic memories) last about 0.5 seconds longer than the actual stimulus. In contrast, auditory sensory memories (echoic memories) last about 3 seconds longer than the actual stimulus. This brief persistence of sensory information in our memory allows us to perceive the world in a continuous and coherent fashion. For example, as we move our eyes from one location to another, we create overlapping sensory memories. The persistence of visual memory can be demonstrated by moving a flashlight or sparkler very quickly to form a shape. For example, if we move our sparkler very quickly in a circular motion, we can often see a circle (see Figure 7.4). This is because the sensory memory of the starting position and all the other intermediate positions of the sparkler are still present by the time we finish drawing the shape. Similarly, sounds stay in our echoic memory for a few seconds allowing us to integrate them with other sounds, such as other syllables in a word, or words in a sentence. Researchers believe that although the duration of sensory memory is brief, it can hold a massive amount of information at one time—in other words, it has a very large capacity.
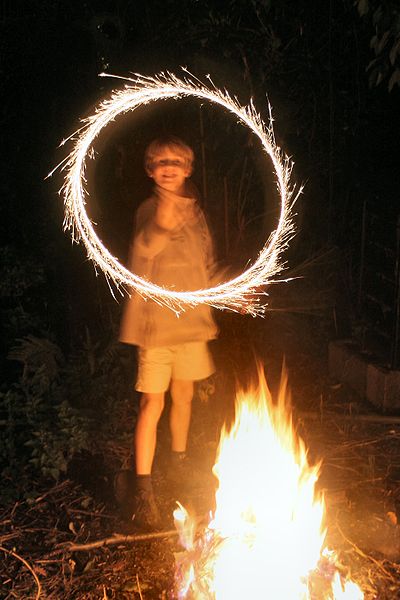
Short-Term Memory/Working Memory
As you know from Chapter 5 when you read about inattentional blindness, we need to pay attention (at least at some level) for information to enter into our conscious thoughts. Otherwise the information is lost. This ensures that we do not waste time processing irrelevant stimuli in our environment. Provided we pay sufficient attention to it, information will make its way into our intermediate memory store. Psychologists use different terms to describe this kind of memory, including short-term memory (STM) and more recently, working memory (WM). Baddeley and Hitch (1974, 2000) proposed that WM is a more accurate description of what happens between sensory and LTM. Working memory describes whatever we are thinking about in a given moment—in other words, what our minds are working on. As you can see in the model in Figure 7.3, information can enter working memory either from LTM (what we already know) or from sensory memory (what is happening in our environment at a given moment).
Because our cultural values influence how we prioritize different kinds of information, they also determine how we direct our attention. Our attentional focus in turn determines how much (and what kinds of information) we remember. For example, people from East Asian countries are more likely to pay equal attention to foreground and background objects in a scene, and so not surprisingly, they have better memory for contextual details and non-focal objects compared to Euro-Americans who attend more to central objects (Wang, 2021).
WM/STM has limited processing abilities. This is why it is so difficult for us to multi-task. For example, taking notes in class can be very demanding, especially if your instructor is talking very quickly about a topic that you have never heard about before. Usually psychologists test WM/STM with simple tasks like asking people to repeat back a sequence of numbers in the same order. There is a limit to the number of digits that we can remember in this kind of task. Test out your working memory using the demonstration below:
Exercise: LET’S TEST YOUR WORKING MEMORY

How did you do? In his much cited article, Miller (1956) estimated the capacity of working memory to be 7 (plus or minus 2), and referred to this as the “magic number”. In other words, most of us are limited to holding between 5 and 9 digits in our conscious thoughts at any one time. However, we can potentially remember more than 7 numbers, if we divide the number string into parts—a strategy known as chunking. For example, a telephone number is typically chunked into three parts, e.g., 212-237-8000. More recent research suggests that we can remember 4 (plus or minus 1) chunks of information (Bodie et al., 2006; Cowan, 2010; Mathy & Feldman, 2012). In the STM/WM test you just did, chunking the numbers into groups of three can be helpful for remembering more of the number string, e.g., 719-384-273. Did you use that strategy? Apart from telephone numbers, can you think of other numbers in our daily lives that are also chunked to help us to remember them? Chunking might help you remember other important numbers that are in a continuous string– like your student ID number.
In addition to the limited capacity of working memory, we can only hold incoming information in our minds for a limited amount of time (about 15 to 30 seconds). For example, if someone tells you a string of numbers (like a phone number) you will probably be able to repeat them back to them in the same order straight away, especially if you use chunking. However, if they give you a distracting task to do first—like counting backwards in 3s from 99, by the time you get to zero, it is unlikely that you will still be able to recite the numbers correctly. The information has been lost. If we want to keep the numbers in STM/WM we need to repeat them – either out loud or silently to ourselves—this is called maintenance rehearsal. However, the distraction of having to count backwards prevents us from rehearsing.
Peterson and Peterson (1959) used a similar technique to investigate how long we can hold new information in working memory, without rehearsing or using other memory strategies. They asked participants to try and remember a list of trigrams. Trigrams are 3-letter nonsense words (e.g., CLS, TPQ etc.) that are used a lot in memory research, because they are unfamiliar to participants. However, they also used distracting tasks of various lengths of time to prevent rehearsal. Participants remembered about 80% of the trigrams after a 3-second delay, but only 10% after a delay of 18 seconds. Therefore, they concluded that a lot of the content of working memory decayed in about 18 seconds (see Figure 7.6. It is also likely that trying to remember the earlier trigrams interfered with the ability to remember later ones—interference is a common issue in memory (Keppel & Underwood, 1962).
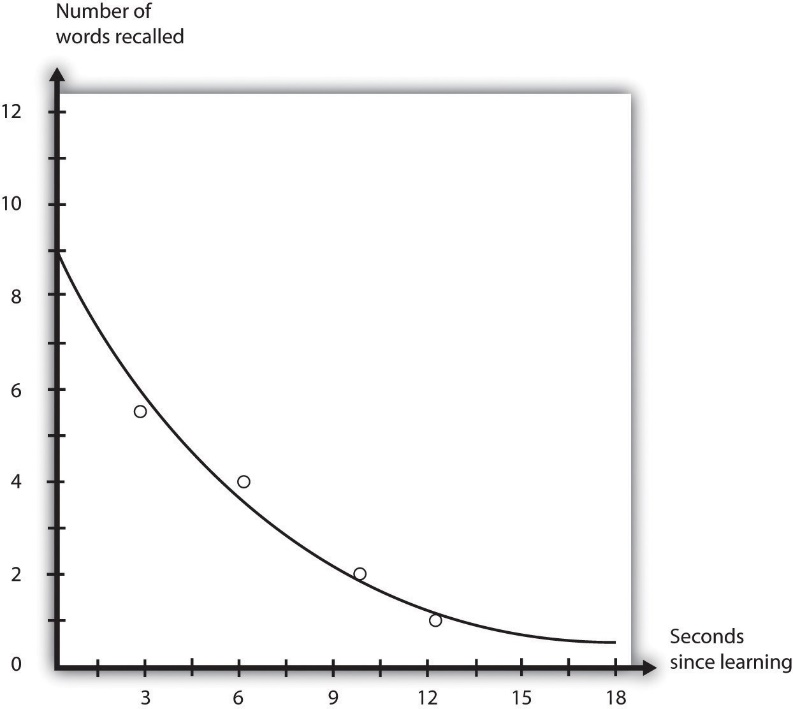
According to Baddeley (2000), we process information in working memory within separate systems (Figure 7.7). There is a visuospatial sketchpad, which processes visual information from the world around us– such as shapes and colors of objects, as well as their location and movement. In contrast, the phonological loop processes sound-related information, such as words that we hear or read. Therefore, our working memory’s “contents” often consists of pictures and internal dialog. Another system, the episodic buffer keeps track of the timing and order of events, and helps to integrate the information from all the different systems. These three systems are controlled by the central executive (like the main boss). The central executive directs our attention towards different kinds of information in our environments and helps us to choose appropriate encoding strategies to move new information into more permanent LTM storage. As you can see in Figure 7.7, information flows between the systems, thus, at any given moment we can pay attention to what is around us, but we can also retrieve existing knowledge from LTM to help us to organize and encode information.
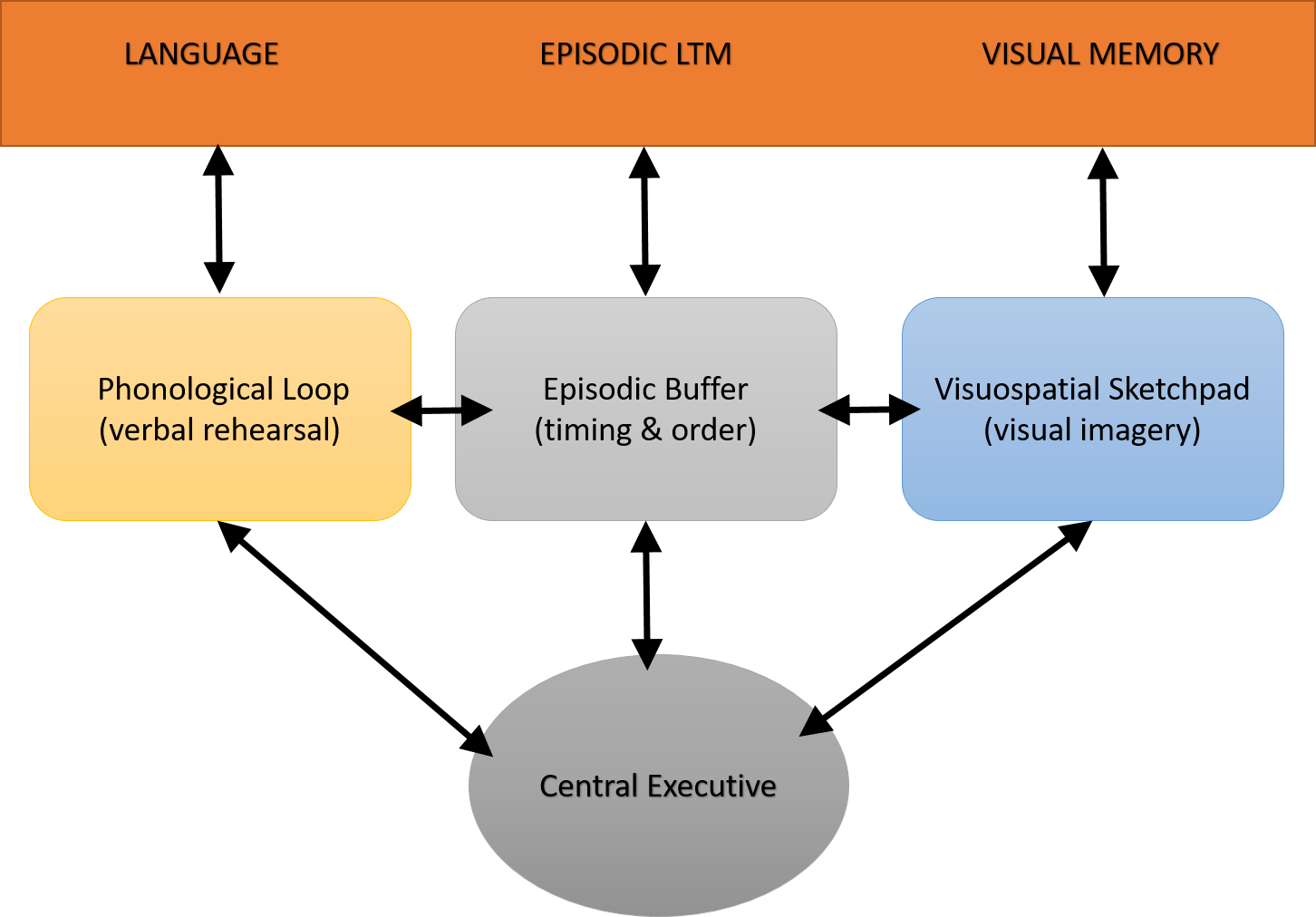
Encoding Information for Long-term Memory
We often want to move information into LTM, so that we can remember it for long periods of time. One strategy is to use simple maintenance rehearsal by repeating the information over and over. This is also known as rote learning. You might have learned your alphabet like this, or possibly your times table. However, it is not the most effective way to learn most information. Let’s take a look at other encoding strategies that have been shown to be more effective—deep processing and semantic encoding. Semantic encoding refers to processing the meaning of the word and making associations with other concepts we already know. These techniques are sometimes referred to as elaborative techniques. Although these strategies are more effortful than rote learning they are more efficient and effective.
Deep processing requires that we pay close attention to information and try to encode it in meaningful ways. Research by Paivio (1986) shows that information that is encoded using both auditory and visual systems of working memory (Figure 7.7) are more easily remembered than information processed from only one system alone. This explains why it is easier to remember concrete words like car, dog, and book, than abstract words such as level, truth, and value. We can easily form mental images of concrete words, but not abstract ones. When we can think about the picture, as well as the sound of the word, it helps us to build a stronger memory (Paivio, 1986).
Craik and Tulving used a different approach to show that deep processing resulted in better memory for words, than shallow processing (Craik & Lockhart, 1972; Craik & Tulving, 1975). Craik and Tulving showed participants a list of words. For each word, the participants were asked one of three questions about them. 1) Was the word written in upper or lower case (visual encoding)? 2) Did the word rhyme with a second word on the screen (auditory encoding)? or 3) Did the word fit in a specific sentence (semantic encoding)? After seeing all the words, participants were given a surprise memory test. Participants remembered the words that they encoded semantically, better than those they encoded visually or acoustically. Semantic encoding involves a deeper level of processing than the visual or acoustic encoding, because participants have to elaborate further to think about the meaning of the word.
You probably already know about the benefits of using semantic encoding when trying to remember new information. It is easiest for us to learn concepts if they make sense to us. You have probably had the experience of memorizing a passage of text—such as a speech, poem, or the lyrics of a song at some point. Was it difficult to do? Some kinds of text are easier to remember than others. Which of the two quotes below do you think would be easier for you to remember and why?
“O, reason not the need!
Our basest beggars
Are in the poorest thing superfluous.
Allow not nature more than nature needs,
Man’s life’s as cheap as beast’s.”
From Shakespeare’s play “King Lear” (Act 2, Scene 4)
“You may shoot me with your words,
You may cut me with your eyes,
You may kill me with your hatefulness,
But still, like air, I’ll rise.”
From Still I Rise by Maya Angelou
Multiple studies have shown that sentences are easier to recall if they are meaningful to us (Anderson, 1984). So, whichever of the two quotes made more sense to you, is the one that you are most likely to remember. For most of us, it’s probably the quote by Maya Angelou. Try out this adaptation of a study by Bransford and McCarrell (1974), which provided scientific evidence showing that meaning is important when trying to learn sentences.
Exercise: READ THESE SENTENCES
- The child was hungry until they broke it.
- The street was full of holes because the turning stopped.
- The woman saw her face in the body.
Now look away and count backwards from 30 in threes (30, 27, 24, etc.) all the way to zero.
Then try to write down the sentences, word for word (no peeking back at this page!).
How well did you do? The statements above do not make a lot of sense and so they were probably difficult for you to remember accurately. Here are some prompts for each sentence that should help them make more sense to you: #1—an egg; #2 —a cement mixer; and #3— a new car. Read the sentences out loud again, keeping the prompts in mind. Next, count backwards from 40 by fours, then check yourself to see how well you recall the sentences this time. You probably did better than before. Of course, you now have had the benefits of already studying the sentences once before, and we know that practice helps information to be retained. But, the sentences are also probably much more memorable because each one was placed in context. This is another example that shows that material is more easily encoded when you make it meaningful.
Neuroscientists believe that concepts in memory are represented within nodes, which are networks of interconnected neurons (Collins & Loftus, 1975). We form semantic networks by building connections between nodes for concepts that are related (Figure 7.8). Activating any part of a semantic network also activates other related concepts, which makes them easier to access and remember. These semantic networks differ from person to person depending on personal experiences and so they are shaped by cultural values.
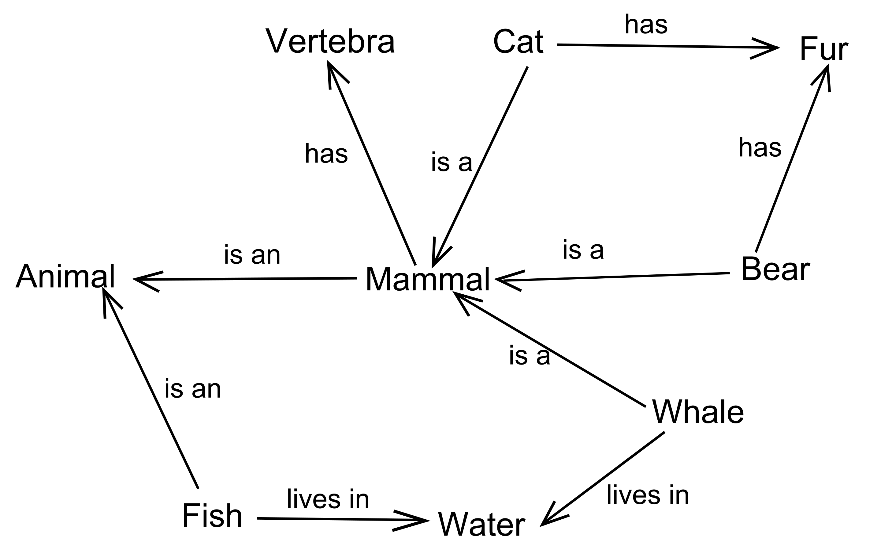
Semantic encoding strategies vary across cultures. When trying to remember lists of words, American participants often automatically organize them in terms of semantic categories. For example, Bousfield (1953) asked American participants to memorize a list of 60 words, which belonged to four different categories (animals, names, jobs, and vegetables). Although the words were presented in random order and the participants were not told about the categories, participants tended to recall them in category clusters, such as a group of animals first and then a group of names. In contrast, when East Asian participants were asked to recall words in a similar kind of task, they used a different semantic encoding strategy. They were more likely to cluster objects thematically in terms of their perceptual and functional similarities, or by associations. For example, they might remember dog, bone, and kennel together in one cluster, and long objects, such as river, serpent, and broom, together in another cluster (Wang, 2021).
Multiple studies with Western participants have shown that people are also more likely to remember information that they feel a personal connection to—such as adjectives that describe themselves—this is known as the self-reference effect (Rogers et al.,1977). However, recent studies suggest that this effect is specific to people from cultures that endorse individualism. In contrast, people from more collectivistic cultures are more likely to remember words relating to relationships, such as roommate or brother (Wang, 2021).
We will return to the importance of semantic encoding at the end of this chapter when we discuss effective learning strategies.
Subdivisions of Long-Term Memory
Although our goal is often to get information into long-term memory, there are several types of biologically distinct LTM (Figure 7.9). All LTMs are divided into explicit (requiring conscious effort for recall) or implicit (recalled automatically) memories. For example, if you are studying for your psychology exam, the material you are learning will (hopefully) become an explicit memory. Explicit memory is sometimes referred to as declarative memory, because it can be “declared” or put into words. Explicit memory is further subdivided into semantic and episodic memory.
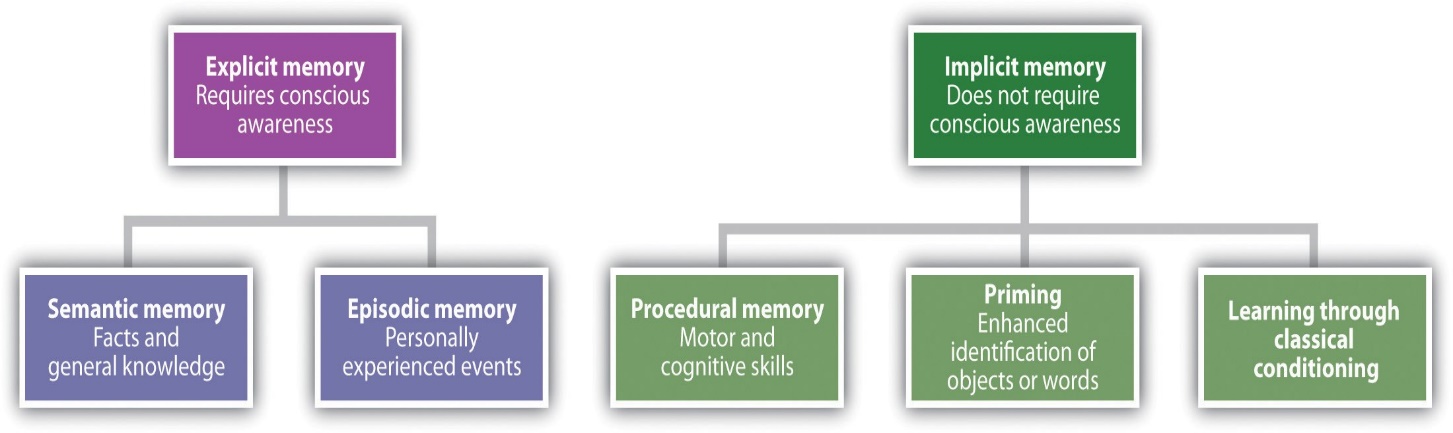
Link to Learning
View this video that explains short-term and long-term memory to learn more about how memories are stored and retrieved.
Explicit Memory
Semantic memory encompasses knowledge about words, concepts, and language-based knowledge and facts. Semantic memory is typically reported as “facts”. In contrast, episodic memory, which is also known as autobiographical memory, includes details about events we have personally experienced. We can think of episodic memory as recalling the episodes of our life—a bit like a TV show. Episodic memories help us to remember the “what, where, and when” of an event (Tulving, 1972; 2002). Episodic memories often involve visual imagery and are typically in story form (Hassabis & Maguire, 2007). The content of episodic memories is also driven by cultural values. For example, Chinese people often value low-arousal harmonic relationships among members of their community, and are more likely to recall episodic memories focusing on loved ones and social interactions. In contrast, people from individualistic cultures typically remember more unique personal details in their stories. The kinds of events most likely to be remembered also vary across cultures, Chinese people are more likely to focus on peaceful occasions and achievements, whereas people from Brazil and Portugal often recall rites of passage more easily (Wang, 2021).
Implicit Memories
Implicit memories are long-term memories that we recall without conscious effort—in other words, we do not summon them—they happen automatically. They include memories learned through classical conditioning and procedural memories, which are actions that once learned become automatic behaviors—such as riding a bike or brushing our teeth. Priming is another example of implicit memory.
Implicit procedural memory stores information about physical actions needed for complex behaviors, such as brushing your teeth, riding a bicycle, typing on a computer keyboard, or driving a car (Adams, 1957; Lacey & Smith, 1954; Lazarus & McCleary, 1951). Even walking and talking are procedural memories. Procedural memories are sometimes referred to as “muscle memories”—we can only learn them through physically doing something with our bodies. Procedural memories are formed through repetition and practice, and once learned they allow behaviors to occur automatically without effort or conscious thought. We often see toddlers struggling to balance and having a hard time coordinating their body movements when they take their first steps, but before long, walking becomes automatic and easy. Similarly, when people first learn to drive, they typically think a lot about how to operate the car – where to put their hands on the steering wheel, which foot to use for the brake and the accelerator, and the meanings of the traffic signs and signals. Learner drivers gradually shift from explicit, conscious thinking to implicit, automatic actions. The actions become streamlined, requiring less cognitive effort and allowing the driver to focus on higher-level tasks such as navigation and other kinds of decision-making.
Priming is another type of implicit memory (Schacter, 1992). If you remember from chapter 5, we say that priming has occurred if exposure to a stimulus influences subsequent thoughts, perceptions, or behaviors, without conscious awareness. Priming stimuli may include single words, sentences or short stories, pictures, videos, and sounds, etc. Priming stimuli can affect our perceptual set and bias the focus of our attention or expectations, without us realizing. Let’s see whether this example of priming affects you.
Priming Exercise
Some people really enjoy picnics. They love going to new places, spreading a blanket on the ground, and eating a delicious meal.
Now, unscramble the following letters to make a word: AETPL
What word did you come up with? Was it “plate?” If you had read a different priming story – such as “Some people really enjoy growing flowers. They love going outside to their garden, fertilizing their plants, and watering their flowers,” you might have come up with the word “petal” instead of “plate”.
The reason that people are more likely to come up with the word “plate” after reading about a picnic is that “plate” and “picnic” are semantically related. Similarly, reading about flowers activates other related concepts within that semantic network – such as “petal”. Therefore, “picnic” primes “plate” and “flower” primes “petal”.
Memories that are formed through classical conditions are a third type of implicit memory. These memories are typically formed and recalled without conscious awareness. For example, Pavlov’s dogs starting to salivate when they heard a bell that was rung prior to them being fed is an example of an implicit memory. Pavlov’s dogs did not consciously have to learn the association between the bell and the food – it was effortless – similarly, their salivation to the bell was also automatic. Humans also have classically conditioned memories, as we discussed in Chapter 6. For example, fear conditioning helps us to avoid places and activities that we have learned were harmful to us, and taste aversion helps us to avoid foods that make us sick.
Everyday Connection
Can You Remember Everything You Ever Did or Said?
What were you wearing exactly five years ago today? What did you eat for lunch on April 10, 2018? You probably find it difficult, if not impossible, to answer these questions about your episodic memory. However, there are a few individuals who have amazing and highly superior autobiographical memory – or hyperthymesia. They are often able to remember every event they have experienced over the course of their life—meals, conversations, clothing choices, weather conditions, and so on. As of 2022, around 50 people have been identified as having this hyperthymesia, but not all have been studied (Parker et al., 2006; University of California Irvine, 2022).
Link to Learning
Watch this video about superior autobiographical memory from the television news show 60 Minutes to learn more.