Chapter 5. Sensation and Perception
5.5. The Other Senses
Vision (our most highly developed sense) and hearing have received an incredible amount of attention from researchers over the years. While there is still more to be learned about how these sensory systems work, we have a much better understanding of them than of our other sensory modalities. In this section, we will explore our chemical senses (taste and smell) and our body senses (touch, temperature, pain, balance, and body position).
Chemical Senses
Taste (gustation) and smell (olfaction) are called chemical senses because both have sensory receptors that respond to chemicals in the food we eat or in the air we breathe. These senses help promote appetite for edible foods and warn us when something is potentially harmful. There is a prominent interaction between our chemical senses. When we describe the flavor of a given food, we are really referring to the interaction between our gustatory and olfactory processes. This helps to explain why food loses its flavor when we have a blocked nose and cannot smell it.
Taste (Gustation)
You may have learned in grade school that there are four basic categories of taste: sweet, salty, sour, and bitter. However, we probably have at least five categories of taste receptors in the mouth and throat. Umami was the fifth taste category to be discovered. Umami is a Japanese word that roughly translates to “yummy”, and it is associated with the savory taste found in foods like meat, cheese, and mushrooms (Kinnamon & Vandenbeuch, 2009).
Taste receptors cells are grouped inside of taste buds; taste receptor cells have hair-like extensions called cilia that poke through the central pore of the taste bud into the saliva. Chemicals in the saliva bind to the receptors on the cilia (Figure 5.27). Taste receptors are found on the tongue, mouth and throat, and have a life cycle of ten days to two weeks, so burning your tongue won’t have any long-term effect; they just grow right back. We have different taste receptors for each of the five basic tastes. When a chemical binds to a receptor, it produces action potentials, which are transmitted to the brain via one of three different nerves, depending on where the receptor is located. Taste information is transmitted to the brainstem, thalamus, and limbic system, and then to the primary gustatory cortex, which is tucked underneath the overlap between the frontal and temporal lobes (Maffei et al., 2012; Roper, 2013). Information is then sent to a multi-sensory area in the brain called the orbitofrontal cortex (OFC), which is responsible for the perception of flavor. The OFC also receives visual, somatosensory, and olfactory information, all of which influence our perception of flavor and whether we are enjoying what we are eating.
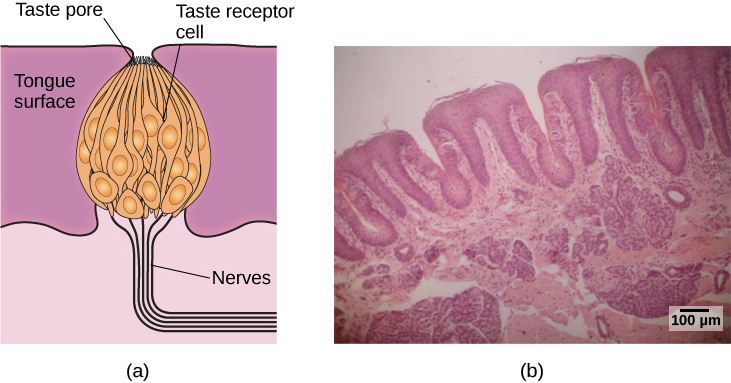
Smell (Olfaction)
Olfactory receptor cells are located in the mucous membrane inside the top of the nose. Chemicals from odorants dissolve in the nasal mucous and stimulate the olfactory receptors that sit on cilia of the olfactory receptor cells (Figure 5.28). Once an odor molecule binds to a given receptor, action potentials are sent to the olfactory bulb: a subcortical structure which sits under the frontal lobe. From there, information is sent to regions of the limbic system and to the primary olfactory cortex, which is located very near to the primary gustatory cortex (Lodovichi & Belluscio, 2012; Spors et al., 2013). Information is then also sent to the OFC.
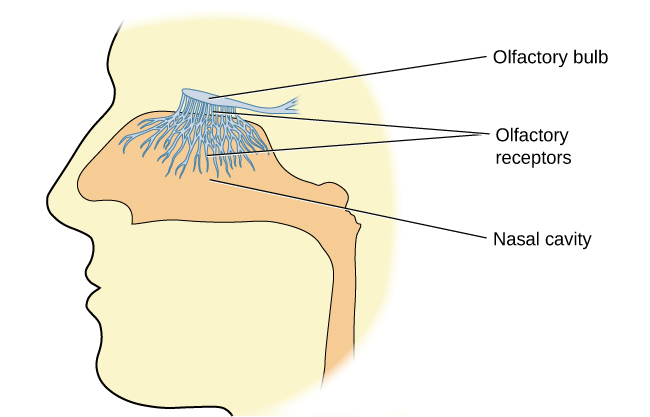
There is tremendous variation in the sensitivity of the olfactory systems across different species. In general, dogs have far superior olfactory systems than our own and are better at detecting most smells than humans. However, there are actually a few odors (like bananas) that we can detect better than dogs (Handwerk, 2017). Some dogs can do some remarkable things with their noses. There is some evidence to suggest that dogs can “smell” dangerous changes in blood glucose levels in people with diabetes, and can detect the presence of cancerous tumors (Wells, 2010). Dogs’ extraordinary olfactory abilities are due to having greater numbers and more types of olfactory receptors compared to humans. It is estimated that dogs have about 220 million olfactory receptors consisting of 800 –1200 different types, whereas humans have about 5 million receptors with fewer than 400 different types (Lippi & Cervellin, 2012; Niimura & Nei, 2007).
Many species respond both behaviorally and physiologically to chemical messages, known as pheromones, that are secreted by other individuals (Wysocki & Preti, 2004). Pheromonal communication often provides information about the reproductive status of a potential mate. For example, when a female rat is fertile, she secretes pheromonal signals that elicit sexual behavior in the male rat (Furlow, 1996, 2012; Purvis & Haynes, 1972; Sachs, 1997). Studies show that humans can also detect odors related to reproduction, which helps to explain why women living together tend to have synchronized menstrual cycles (Cherry & Baum, 2020; Miller & Maner, 2010; Stern & McClintock, 1998). The vomeronasal organ in the nose, which detects pheromones, is very small in humans, which has cast some doubt as to whether these phenomena are actually mediated by pheromones. However, alternative neural pathways for processing pheromones have recently been suggested (Cherry & Baum, 2020).
Touch, Thermoception, and Nociception
In Chapter 3, you read about the primary somatosensory cortex in the parietal lobe, which receives information about touch from different parts of the body. However, there are many different aspects of body sensation, including heat and pain detection. We have different somatosensory receptors at different levels of the skin, which respond to specific aspects of stimuli in different ways (Figure 5.29). For example, both Merkel’s disks and Meissner’s corpuscles are present in the surface of the skin and respond to light touch, but in different ways. Meissner’s disks respond to changes in touch, whereas Merkel’s disks respond to sustained touch. So, if a small insect hops onto our hand, the Meissner’s corpuscles in that part of the skin will respond, but they stop responding if the insect stays still. However, Merkel’s disks let you know that the insect is still there. Pacinian corpuscles are found deeper in the skin, so respond to more intense pressure, like a small poke. However, they too only respond to changes in touch, i.e., when the poking begins or ends. Ruffini corpuscles are present in the collagen, deep in the skin and respond in a sustained way to stretch (Abraira & Ginty, 2013). All of these receptors are called mechanoreceptors because they undergo physical movement when they are stimulated, which is converted into neural energy and sent to the brain to be processed.
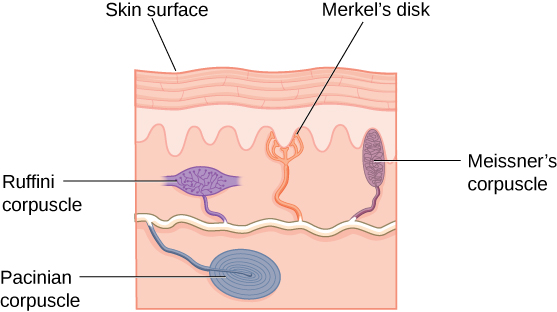
We also have proprioceptors in our joints, muscles, and tendons that send information about the position and movement of our body to our brain (Lackner & DiZio, 2005; Proske, 2006; Proske & Gandevia, 2012). This information travels via the spinal column, to the cerebellum and other parts of the brain. These receptors allow us to perceive the relative position of our body (proprioception), which is important for our sense of balance. They also contribute to our awareness of how our bodies are moving (kinesthesia).
There are also a number of free nerve endings in the skin that respond to thermoception (temperature perception) and nociception, which are the unpleasant sensations that can be elicited by stimuli that are too hot or too cold, or by excessive pressure (Garland, 2012; Petho & Reeh, 2012; Spray, 1986). All signals from the body’s sense receptors are sent to the primary somatosensory cortex via the pons and thalamus.
Pain Perception
Pain is an unpleasant experience that involves both physical and psychological components. Feeling pain is adaptive because it makes us aware of an injury, and it motivates us to move away from the cause of that injury. In addition, pain also makes us less likely to suffer additional injury because we are typically more careful with our injured body parts.
Nociceptors provide information about transient threats, such as briefly touching a hot stove. But, they also respond to more prolonged pain that can arise from inflammation (tissue damage) or neuropathy (peripheral or central nervous system damage). In neuropathy, pain signals that are sent to the brain are amplified resulting in intense and often chronic pain. The unpleasantness of pain is an emotional response, which activates the limbic system, and in particular, the anterior cingulate cortex (ACC). Multiple treatment options for pain relief range from relaxation therapy to the use of analgesic medications to deep brain stimulation. The most effective treatment option for a given individual will depend on a number of considerations, including the severity and persistence of the pain and any medical/psychological conditions. Pain relief reduces activation in the ACC. In the previous chapter on consciousness, we talked about how hypnosis can reduce ACC activity and alleviate pain.
Some individuals are born without the ability to feel pain, a condition known as congenital analgesia. This is a very rare genetic disorder, where people can detect differences in temperature and pressure, but they cannot experience pain. As a result, they often suffer significant injuries. Young children suffer serious mouth and tongue injuries because they have bitten themselves repeatedly. Not surprisingly, individuals with this disorder have much shorter life expectancies due to more injuries and related secondary infections (U.S. National Library of Medicine, 2013).
Link to Learning
Watch this video about congenital insensitivity to pain to learn more.
The Vestibular Sense, Proprioception, and Kinesthesia
The vestibular sense contributes to our ability to maintain balance and body posture. As seen in Figure 5.30, the major vestibular organs (utricle, saccule, and the three semicircular canals) are located in the inner ear. The vestibular organs are fluid-filled and have hair cells, similar to the ones found in the auditory system. These respond to movement of the head and gravitational forces. When these hair cells are stimulated, they send signals to the brain via the vestibular nerve. Although we may not be consciously aware of our vestibular system sending information under normal circumstances, we can experience unpleasant symptoms such as motion sickness and/or dizziness when the vestibular system is stimulated by movement or when we have infections of the inner ear (Khan & Chang, 2013). In the brain, information from the vestibular system also interacts with that coming from proprioceptors, which is used to help control body position.
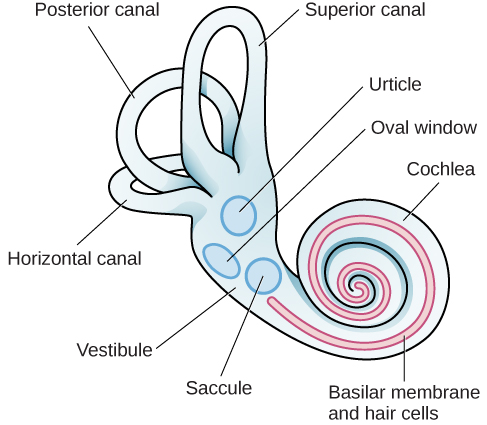
Multimodal Perception
In this chapter we have largely considered our senses as separate from each other, because this is mostly how they have been studied. However, real-life perception mostly depends on information from multiple senses interacting at the same time. Imagine a having special meal at a restaurant – you experience the smell, taste, texture, temperature and flavor of the food, but also the ambience in terms of the lighting, décor, music, and the loudness of the diners around you. Your dining experience – like most real-life experiences—is multi-modal, it clearly depends on all of your senses. The brain usually processes multi-modal information more efficiently, than information from just one sensory system. We have specialized neurons in multiple parts of our brain that allow us to integrate multimodal sensory information. We have already mentioned the OFC – a multisensory brain area that is important for the perception of flavor. Another multisensory cortical area is the superior temporal sulcus. The superior temporal sulcus has neurons that respond to both visual and auditory stimuli, which are particularly helpful in understanding speech. Watching the movements of people’s mouths helps us to understand what they are saying – especially in a noisy environment. Even subcortical areas like the superior colliculus in the midbrain have multisensory neurons. These allow us to rapidly respond to sudden loud sounds in our environment. Imagine if someone behind you suddenly drops a glass and it crashes to the floor. The sound rapidly activates the neurons in the superior colliculi, which also enable us to move our heads quickly and use our eyes in combination with our hearing to figure out whether we are in danger. This is considered to be an automatic orientation response, that helps to protect us from potential threats.