46 Earthquake Hazards and Prediction
Earthquakes can be hazardous to humans and property in a variety of ways.
Earthquake hazards arise from a combination of factors such as the size of the earthquake, distance to the epicenter, the underlying material and geologic structures, and building construction.
Potential Hazards
Ground Shaking
Ground shaking is caused by seismic waves. During a significant earthquake, a particular location, and any building at that location, will be shaken by body waves (P- and S-) and surface waves (R- and L-). Each type of wave will have a different frequency (different number of waves passing by each second), and can shear or move a building in a different way, sometimes simultaneously. Adjacent neighborhoods or towns may experience very different intensities from the same earthquake, based on how far they are from the epicenter and what sort of rocks or sediments are in the ground beneath each area.
Places underlain by thick deposits of unconsolidated sediments will experience a higher amplitude of shaking, at the same distance from the same earthquake, than places underlain by solid bedrock all the way to the surface. If unconsolidated sediments are fine-grained and wet, they may undergo liquefaction, increasing the damage to buildings and infrastructure and therefore increasing the intensity of the earthquake there.
If unconsolidated sediments are overlain by a layer of artificial fill, the area is likely to experience more intense shaking and undergo more ground settling, and liquefaction if wet, than places that have not had a layer of artificial fill added.
Mexico City, one of the most populated cities in the world, is in a basin in the mountains of Mexico. Much of the city is built on artificial fill on top of fine-grained sediments from an extensive lake and wetlands that were drained and filled in as the city grew. As a result of how seismic waves are amplified in soft sediments, the shaking of the ground in Mexico City during an earthquake is greater than it is in areas outside the basin, which have bedrock close to the surface. During the 1985 earthquake, which originated offshore of Acapulco on the Pacific coast, 300 km (200 miles) away, many buildings in Mexico City collapsed and more than 20,000 people died.
The Marina District in northern San Francisco is built on artificial fill on wet bayshore sediments. Rubble and debris from buildings that collapsed or burned in that district as a result of the 1906 earthquake was used as artificial fill beneath structures built during the reconstruction of that area. In 1989, the Loma Prieta earthquake caused collapse of several buildings in the Marina District, and several people died there. Another example of a structure on wet ground covered by artificial fill that collapsed during the Loma Prieta earthquake is the Cypress viaduct in Oakland, CA. The USGS photo below shows the failed support columns.
Permanent Ground Displacement
During large earthquakes, the ground may permanently shift to a new position up, down, or sideways (up to 10 or more m, 30 or 40 ft, in extreme cases). This change in the location of the ground, which also tilts the ground, may cause disruption of roads and utilities and, in coastal cities, submergence or emergence of harbor facilities. Even ground shifts of less than a meter (a foot or two) can cause serious disruption to infrastructure.
Earth Rupture
During most earthquakes, some rupturing of the earth’s surface takes place along the fault trace. This produces a fault scarp, which may have up to several m (up to 10 ft or more) of vertical displacement. This can disrupt roads and utilities, and any buildings on a fault that ruptures may undergo extensive damage.
Rupture of the earth during an earthquake may also occur on secondary faults. Earthquake-induced rupture of the earth’s surface may also take place in weak zones of surface sediment that fracture and spread. If enough spreading of a ruptured surface layer takes place, it can be classified as a landslide.
Landslides and Avalanches
On steep slopes and in mountainous areas, large earthquakes can set off many landslides, rockfalls, or avalanches. These can damage buildings, towns, or roads in the path of the landslides.
Liquefaction
If fine- or medium-grained, unconsolidated sediments are saturated with groundwater, the shaking that occurs during an earthquake may cause the sediment grains to lose contact with each other and become suspended in the water, temporarily turning what was solid ground into liquid ground. Building and other structures may sink, tilt, or slide a short distant in liquefied ground, causing serious damage.
Fires
Fires are a secondary rather than a primary effect of earthquakes. Broken electrical wires and natural gas pipes commonly set off fires during earthquakes. To compound the problem, the water supply may also be disrupted by earthquake damage, making it impossible to put the fire out with water from fire hydrants. The fire that broke out as a result of the great San Francisco earthquake of 1906 burned much of the city to the ground, causing more extensive damage to buildings than the shaking of the ground did during the earthquake.
Tsunamis
A tsunami is a set of waves in the ocean (or a large lake) with an extremely long wavelength, typically over 100 km long. Tsunamis move at many 100s of km per hour in deep water. Tsunamis can be set off by violent volcanic eruptions originating just below sea level, by giant landslides that either occur underwater or tumble into the sea from coastal mountains, by large meteorite impacts, and, most commonly, by earthquakes that greatly shake the ocean floor, which commonly happens at subduction zones.
The amplitude, or crest height, of an individual tsunami wave may be only about 1 m (roughly 3 feet high) in the open ocean. It is common for a tsunami to pass ships at sea without being noticed. However, as the wave approaches shore where the bottom grows shallower, the crest builds up to a height of up to several tens of meters (over 30 feet in some cases). The wave crest may wash ashore for several minutes before subsiding. Even a tsunami wave of no higher than 3 m (10 feet) coming ashore can cause extensive damage in harbors and to shores, as the long-wavelength wave keeps pouring in for several minutes.
Tsunamis consist of more than one wave, so a second wave crest may climb ashore several minutes later. Some tsunamis lead with the wave trough, so the first thing noticed as that type of tsunami approaches a shore is a dramatic drawback, or retreat, of the sea, like the tide suddenly going out. Such a drawback will inevitably be followed by a rising tsunami wave crest.
The tsunamis from the Sumatra earthquake of 2004 killed over 100,000 people in coastal areas of the Indian Ocean, some on shores several thousand km (several thousand miles) away from the epicenter of the earthquake. The great southeast Alaska earthquake of 1964 generated a tsunami that killed 16 people on the coast of Oregon and northern California, over 1,000 km (600 miles) away. Tsunamis have been known to cross the entire Pacific Ocean and cause fatalities a third of the way around the world. Hawaii, in the middle of the Pacific Ocean, has been damaged by tsunamis, originating from subduction earthquakes on the Pacific Rim, several times in the last few centuries. Japan, with its complex set of subduction zones and its eastern shoreline open to the Pacific Ocean, has experienced over 100 tsunamis in its recorded history, most recently as a result of a great subduction earthquake off the northern island in 2011 which resulted in several coastal towns being destroyed and thousands of people dying. The last great tsunami generated by the Cascadia subduction zone along the coast of the Pacific Northwest flooded coastal areas of Washington, Oregon northern California, and Vancouver Island in Canada. It occurred in 1701, long before the region was as populated as it is now. Subduction continues in the Cascadia subduction zone and more tsunamis can be expected to be generated by earthquakes there in the future.
Mitigating Earthquake Damage
Much can be done to reduce the risk of fatalities during earthquakes, and to reduce the damage to buildings and infrastructure; in other words, to mitigate the effects of earthquakes.
In many cases, it is collapsed buildings that cause the most harm during an earthquake. Buildings should be constructed in ways that make them unlikely to collapse during an earthquake. The strategies that engineers have developed include having sufficient flexibility in the structure to absorb shaking during an earthquake. Bricks, mortar, and concrete are rigid and brittle. However, bricks and mortar, and concrete, can be reinforced with steel to make them better able to survive an earthquake. Wood and steel are more flexible than bricks, mortar, and concrete, and lend themselves to the type of building that, properly designed and built according to code, is likely to survive an earthquake without collapsing.
The way a building is attached to its foundation, and how the foundation is anchored in the earth, are important considerations in earthquake design. Many houses built in the the early and mid-1900s in California were not attached to their foundations, based on the assumption that the weight of a house would keep it on its foundation. It turned out to be a bad assumption. Earthquakes caused houses to slide off their foundations. Many home-owners in the state have taken steps to make sure that their houses are now attached to their foundations; if a home-owner buys earthquake insurance, it is usually required by the insurance company that they do so. A large building or skyscraper built in an earthquake-prone area will normally have a great deal of flexibility built into it, including some sort of elastic strain absorption mechanism focused on points where the building attaches to its foundation.
Infrastructure—roads, bridges, utilities—can be built with margins of safety for the event of an earthquake. This includes gas pipelines designed to slide back and forth on their supports and having built-in shutoff valves that may be activated by automatic sensors, electric lines and grids with similar flexibility and shut-off capabilities, and roads, overpasses, and bridges built to withstand shaking during an earthquake.
Development and enforcement of building codes aimed at reducing risk from earthquakes often requires resources that are not available in impoverished regions. This leads to a higher likelihood that buildings will collapse from the same size earthquake in some areas of the world than in other areas.
Buildings can be designed to withstand earthquakes: NYT, June 5, 2019
Seismicity and Earthquake Prediction
In the United States, the areas that most frequently experience earthquakes are the coast of California, Oregon, and Washington, the southern coast and Aleutian Islands of Alaska, Hawaii, and the mountain west from the Rocky Mountains to the Pacific coast. The central and eastern United States rarely experience significant earthquakes.
Earthquake epicenters compiled on a map show that, on a global basis, most earthquakes occur around the rim of the Pacific Ocean, in the mountains of southern Asia from China to the Middle East, and in the Mediterranean Sea area. Earthquake epicenters also trace the mid-ocean ridges across the floors of the oceans.
Because nearly all earthquakes occur on faults, determining seismic risks on a finer scale largely consists of identifying, mapping, and studying active faults in each state or region. However, many active faults are hidden, either because any scarps they formed at the surface have been eroded or covered by sediments, soil, and vegetation, or because they are blind faults. A hidden fault is often not identified and located until one or more significant earthquakes has occurred on it and the seismic waves have been studied to determine its location and type of fault motion.
Information used to determine the seismicity of an area includes:
- frequency of earthquakes in the past, as deduced from:
- historic records
- geologic studies that examine evidence of the prehistoric earthquake record
- location of known active faults
- seismologic data collected on recent earthquakes that have occurred in the area
- tectonic setting of the area in terms of proximity to plate boundaries, and information about the plate boundary if one is nearby
- stress and strain being experienced by the crust in that area based on measurements from GPS equipment and from stress and strain measurements conducted in boreholes
- underground geologic layers and structures in that area based on cross-sections from geologic mapping, data from drilling, and remote imaging of deeper layers of the crust and mantle
Based on this information, the seismic risk of a particular area can be quantified statistically. For example, the odds of a major earthquake happening in the next century, or in the next 10 years, can be estimated for a specific seismic zone.
However, no scientific method has yet been developed that can predict precisely when the next earthquake in a specific region will happen, where it will happen, or what its magnitude will be. Scientists have looked into using such possible pre-earthquake indicators as ground tilting, changes in well water levels, changes in radon gas in groundwater near fault zones, changes in electrical conductivity in the earth around faults, changes or patterns in seismic activity that can be measured by seismometers even though it is not felt by humans, and strange animal behavior which, according to numerous, largely unconfirmed anecdotes, takes place before an earthquake. But, so far, none of these types of data have been found to lead to reliable earthquake predictions.
People have also looked into correlations between earthquakes and phases of the Moon, earthquakes and the time of day (such as dawn when the Sun is first shining on the ground), and so on. No connections have been found between earthquakes and these other types of phenomena.
As the study of seismicity stands now, we can identify which areas on earth will undergo major earthquakes in the coming decades and centuries, we can delineate which areas on earth are at risk for the most powerful types of earthquakes, and map the coastal areas that are most at risk of being inundated by a tsunami, but we cannot pinpoint in advance the date or location of the next major earthquake.
Scientists are a long way from being able to predict earthquakes. A good prediction must be accurate as to where an earthquake will occur, when it will occur, and at what magnitude it will be so that people can evacuate. An unnecessary evacuation is expensive and causes people not to believe authorities the next time an evacuation is ordered.
Where an earthquake will occur is the easiest feature to predict. Scientists know that earthquakes take place at plate boundaries and tend to happen where they’ve occurred before (figure 1). Earthquake-prone communities should always be prepared for an earthquake. These communities can implement building codes to make structures earthquake safe.
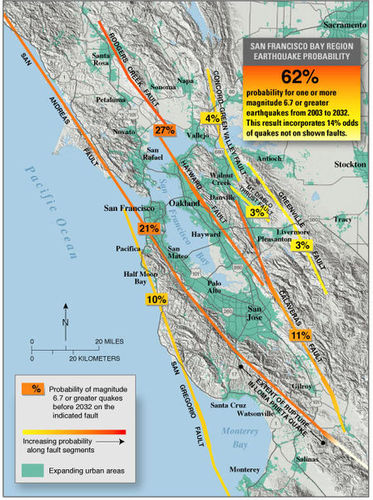
When an earthquake will occur is much more difficult to predict. Since stress on a fault builds up at the same rate over time, earthquakes should occur at regular intervals (figure 2). But so far scientists cannot predict when quakes will occur even to within a few years.
Signs sometimes come before a large earthquake. Small quakes, called foreshocks, sometimes occur a few seconds to a few weeks before a major quake. However, many earthquakes do not have foreshocks and small earthquakes are not necessarily followed by a large earthquake. Often, the rocks around a fault will dilate as microfractures form. Ground tilting, caused by the buildup of stress in the rocks, may precede a large earthquake, but not always. Water levels in wells fluctuate as water moves into or out of fractures before an earthquake. This is also an uncertain predictor of large earthquakes. The relative arrival times of P-waves and S-waves also decreases just before an earthquake occurs.
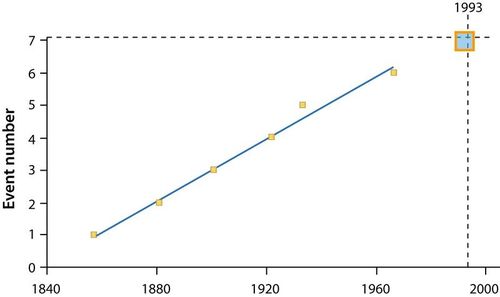