68 Porosity and Permeability, Darcy Law
There are lots of terms in hydrogeology, most of which are very simple, but essential. Here are a few of the big ones and their meanings.
Porosity
Porosity is the amount of empty space in sediments or rocks. In a soil or rock the porosity (empty space) exists between the grains of particles or minerals. In a material like gravel the grains are large and there is lots of empty space between them since they have angularity or spherical shape. However, in a material like a gravel, sand and clay mixture the porosity is much less as the smaller grains fill the spaces.
The amount of water a material can hold is directly related to the porosity since water will try and fill the empty spaces in a material.
We measure porosity by the percentage of empty space that exists within a particular porous media.
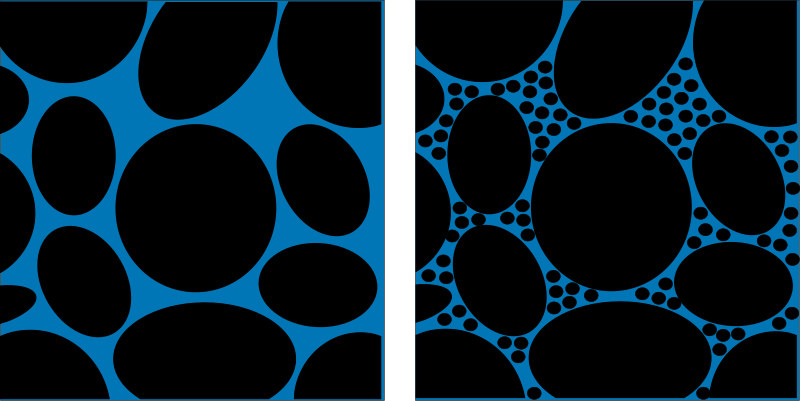
Permeability
Figure 3. Video showing how connected pores have high permeability and can transport water easily. Note that some pores are isolated and cannot transport water trapped within them.
Aquifer
An aquifer is a term for a type of soil or rock that can hold and transfer water that is completely saturated with water. That means that all it is simply a layer of soil or rock that has a reasonably high porosity and permeability that allows it to contain water and transfer it from pore to pore relatively quickly and all of the pore spaces are filled with water.
Good examples of aquifers are glacial till or sandy soils which have both high porosity and high permeability. Aquifers allows us to recover groundwater by pumping quickly and easily. However, overpumping can easily reduce the amount of water in an aquifer and cause it to dry up.
Aquifers are replenished when surface water infiltrates through the ground and refills the pore spaces in the aquifer. This process is called recharge. It is especially important to ensure that recharge is clean and uncontaminated or the entire aquifer could become polluted. There are two main types of aquifers: confined and unconfined. Unconfined is the one connected to the surface and supported by aquitard (impervious surface). Confinded aquifer is located between two aquitards. In this case, the rocks surrounding the aquifer confines the pressure in the porous rock and its water. If a well is drilled into this “pressurized” aquifer, the internal pressure might (depending on the ability of the rock to transport water) be enough to push the water up the well and up to the surface without the aid of a pump, sometimes completely out of the well. This type of well is called artesian.
The rate of recharge is not the same for all aquifers, though, and that must be considered when pumping water from a well. Pumping too much water too fast draws down the water in the aquifer and eventually causes a well to yield less and less water and even run dry. In fact, pumping your well too fast can even cause your neighbor’s well to run dry if you both are pumping from the same aquifer.
In the diagram below, you can see how the ground below the water table (the blue area) is saturated with water. The “unsaturated zone” above the water table (the greenish area) still contains water (after all, plants’ roots live in this area), but it is not totally saturated with water. You can see this in the two drawings at the bottom of the diagram, which show a close-up of how water is stored in between underground rock particles.
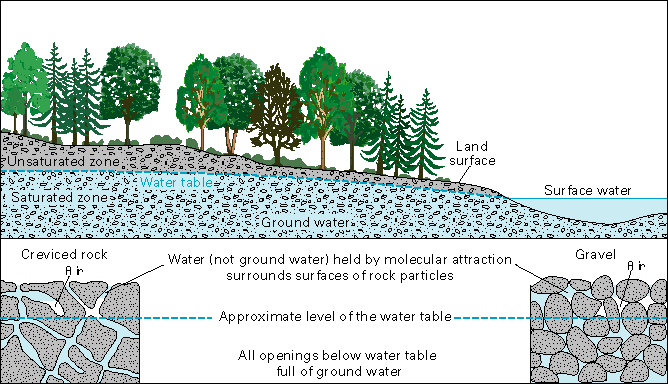
A relationship does not necessarily exist between the water-bearing capacity of rocks and the depth at which they are found. A very dense granite that will yield little or no water to a well may be exposed at the land surface. Conversely, a porous sandstone may lie hundreds or thousands of feet below the land surface and may yield hundreds of gallons per minute of water. Rocks that yield freshwater have been found at depths of more than 6,000 feet, and salty water has come from oil wells at depths of more than 30,000 feet. On the average, however, the porosity and permeability of rocks decrease as their depth below land surface increases; the pores and cracks in rocks at great depths are closed or greatly reduced in size because of the weight of overlying rocks.
Water Movement in Aquifers
Water movement in aquifers is highly dependent of the permeability of the aquifer material. Permeable material contains interconnected cracks or spaces that are both numerous enough and large enough to allow water to move freely. In some permeable materials groundwater may move several metres in a day; in other places, it moves only a few centimeters in a century. Groundwater moves very slowly through relatively impermeable materials such as clay and shale.
After entering an aquifer, water moves slowly toward lower lying places and eventually is discharged from the aquifer from springs, seeps into streams, or is withdrawn from the ground by wells. Groundwater in aquifers between layers of poorly permeable rock, such as clay or shale, may be confined under pressure. If such a confined aquifer is tapped by a well, water will rise above the top of the aquifer and may even flow from the well onto the land surface. Water confined in this way is said to be under artesian pressure, and the aquifer is called an artesian aquifer.
Aquitard
An aquitard is basically the opposite of an aquifer. Aquitards have very low permeability and do not transfer water well at all. In fact, in the ground they often act as a barrier to water flow and separate two aquifers. A good example of an aquitard is a layer of clay. Clay often has high porosity but almost no permeability meaning it is essentially a barrier which water cannot flow through and the water within it is trapped.
Figure below shows low hydraulic conductivity confining unit and very low hydraulic conductivity bedrock unit, both are examples of aquitards.
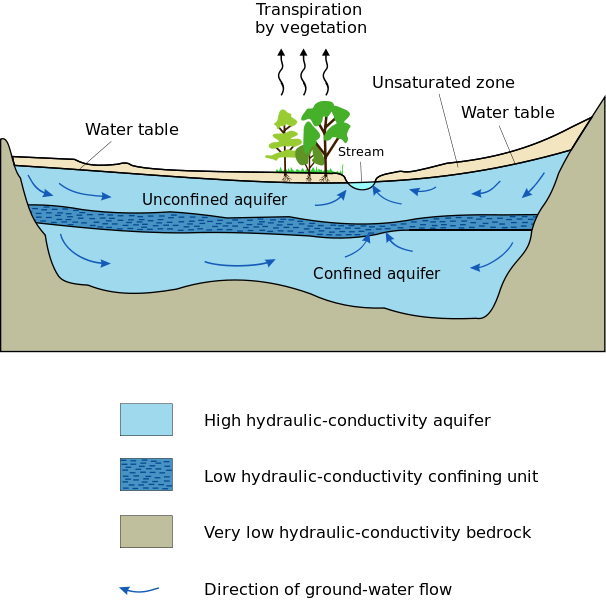
Main Law in Hydrogeology: Darcy’s Experiments and Darcy’s Law
Modified from: https://www.tecquipment.com/knowledge/2019/henry-darcy-of-darcys-law
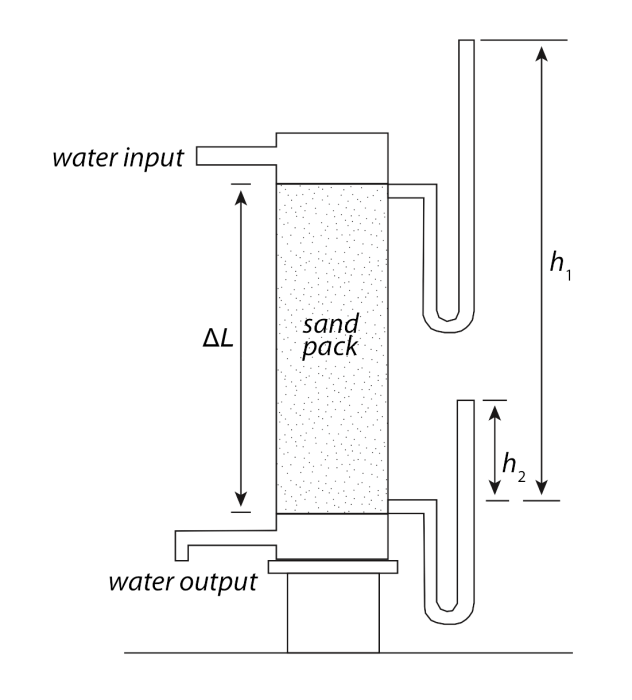
Darcy’s findings laid the foundation for the modern science of hydrogeology by quantifying the relationships between volumetric groundwater flow rate, driving forces, and aquifer properties.
Specifically, Darcy’s experiments revealed proportionalities between the flux of water, Q, through the laboratory “aquifer” and different characteristics of the experimental system (refer to figure above):
(1) Q was directly proportional to the difference in water levels from inlet to outlet, h1 – h2 = Δh:
Q ∝ Δ h
(2) Q was directly proportional to the cross sectional area of the tube:
Q ∝ A
(3) Q was inversely proportional to the length of the column:
Q ∝ 1 / ΔL
Combining these proportionalities leads to Darcy’s Law, the empirical law that describes groundwater flow:
Q = K A (Δh / ΔL)
where K is a constant of proportionality that defines the water flux for a given hydraulic gradient (Δh / ΔL). The above equation can also be recast in terms of the water volume flux per unit area, Q/A (also called “Darcy flux” or “Darcy velocity” with units of length per time):
Q / A = K (Δh / ΔL)
Note that in Darcy’s Law, the other terms all describe the driving forces or geometry of the experimental system; none of them would change if the sand pack in the tube were changed to gravel, silt, or another material. This is where the constant of proportionality, K, comes in: it describes the ability of the material in the column to transmit water (sound familiar?). Indeed, the constant of proportionality and hydraulic conductivity (also K) are one and the same! For example, consider the same experimental geometry, but with silt or clay in the tube rather than sand.
The flow rate would be lower, and this would be described in Darcy’s Law by a smaller value of K.
Darcy’s Law helps to measure K, hydraulic conductivity of soils if we know aquifer length, width, height and quantity of water (Q, discharge). By measuring K we can apply this information for gorundwater modeling in relation to engineering and water supply.